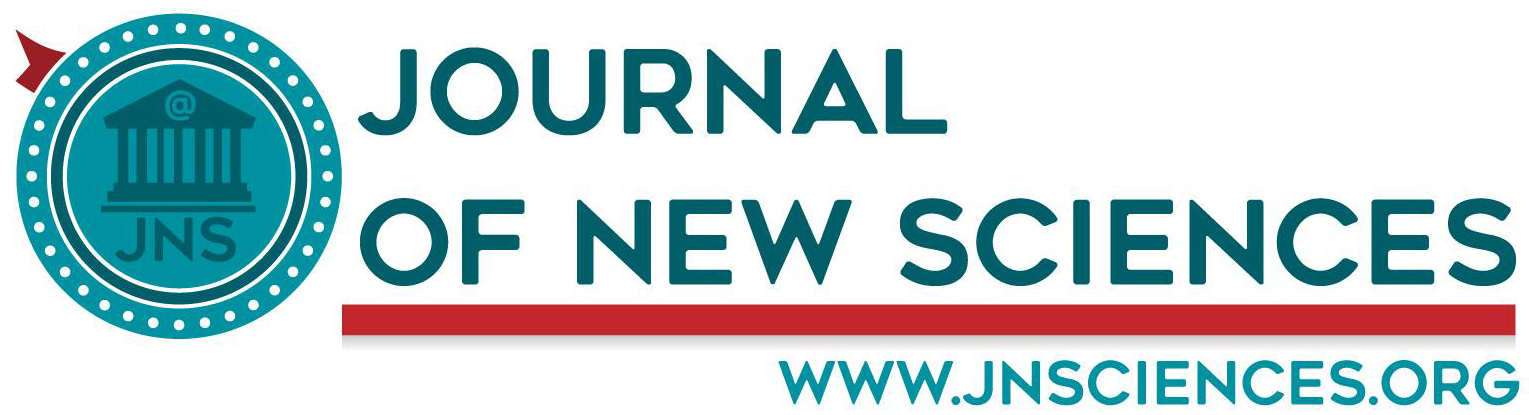
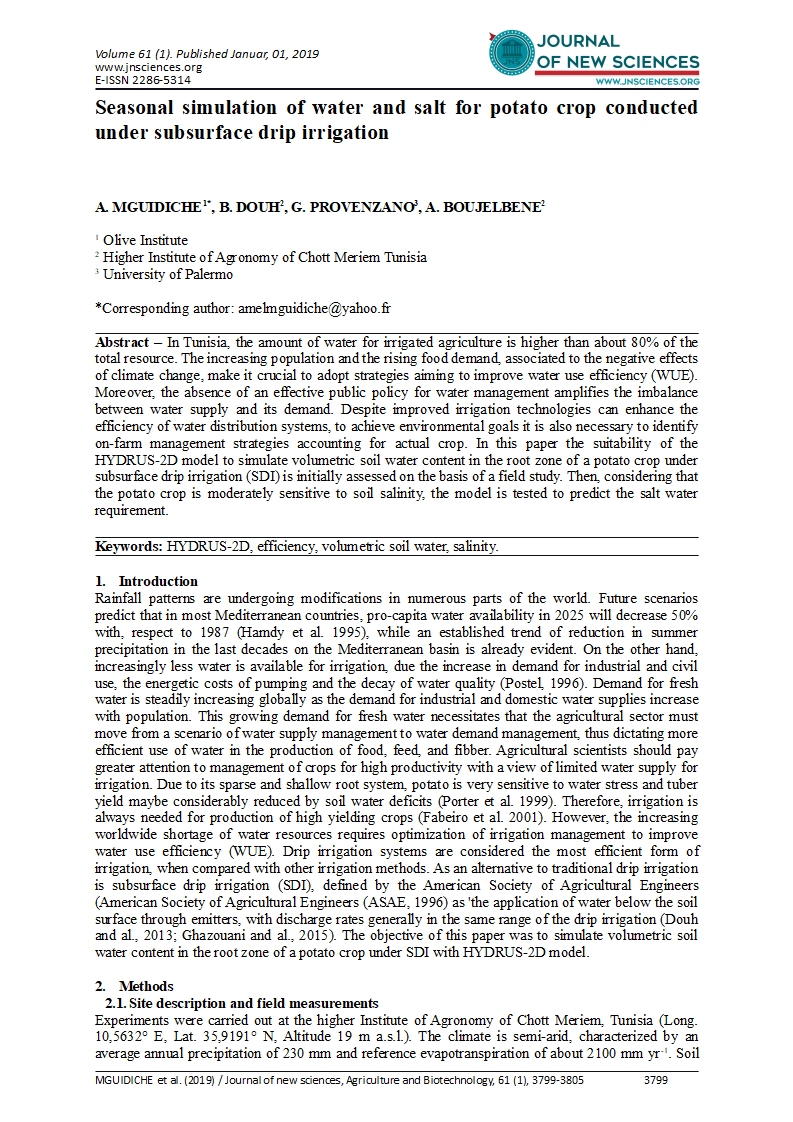
- Category: Volume 61
- Hits: 4322
Seasonal simulation of water and salt for potato crop conducted under subsurface drip irrigation
A. MGUIDICHE1
B. DOUH2
G. PROVENZANO3
A. BOUJELBENE2
1 Olive Institute
2 Higher Institute of Agronomy of Chott Meriem Tunisia
3 University of Palermo
Abstract – In Tunisia, the amount of water for irrigated agriculture is higher than about 80% of the total resource. The increasing population and the rising food demand, associated to the negative effects of climate change, make it crucial to adopt strategies aiming to improve water use efficiency (WUE). Moreover, the absence of an effective public policy for water management amplifies the imbalance between water supply and its demand. Despite improved irrigation technologies can enhance the efficiency of water distribution systems, to achieve environmental goals it is also necessary to identify on-farm management strategies accounting for actual crop. In this paper the suitability of the HYDRUS-2D model to simulate volumetric soil water content in the root zone of a potato crop under subsurface drip irrigation (SDI) is initially assessed on the basis of a field study. Then, considering that the potato crop is moderately sensitive to soil salinity, the model is tested to predict the salt water requirement.
Keywords: HYDRUS-2D, efficiency, volumetric soil water, salinity
1. Introduction
Rainfall patterns are undergoing modifications in numerous parts of the world. Future scenarios predict that in most Mediterranean countries, pro-capita water availability in 2025 will decrease 50% with, respect to 1987 (Hamdy et al. 1995), while an established trend of reduction in summer precipitation in the last decades on the Mediterranean basin is already evident. On the other hand, increasingly less water is available for irrigation, due the increase in demand for industrial and civil use, the energetic costs of pumping and the decay of water quality (Postel, 1996). Demand for fresh water is steadily increasing globally as the demand for industrial and domestic water supplies increase with population. This growing demand for fresh water necessitates that the agricultural sector must move from a scenario of water supply management to water demand management, thus dictating more efficient use of water in the production of food, feed, and fibber. Agricultural scientists should pay greater attention to management of crops for high productivity with a view of limited water supply for irrigation. Due to its sparse and shallow root system, potato is very sensitive to water stress and tuber yield maybe considerably reduced by soil water deficits (Porter et al. 1999). Therefore, irrigation is always needed for production of high yielding crops (Fabeiro et al. 2001). However, the increasing worldwide shortage of water resources requires optimization of irrigation management to improve water use efficiency (WUE). Drip irrigation systems are considered the most efficient form of irrigation, when compared with other irrigation methods. As an alternative to traditional drip irrigation is subsurface drip irrigation (SDI), defined by the American Society of Agricultural Engineers (American Society of Agricultural Engineers (ASAE, 1996) as 'the application of water below the soil surface through emitters, with discharge rates generally in the same range of the drip irrigation (Douh and al., 2013; Ghazouani and al., 2015). The objective of this paper was to simulate volumetric soil water content in the root zone of a potato crop under SDI with HYDRUS-2D model.
2. Methods
2.1. Site description and field measurements
Experiments were carried out at the higher Institute of Agronomy of Chott Meriem, Tunisia (Long. 10,5632° E, Lat. 35,9191° N, Altitude 19 m a.s.l.). The climate is semi-arid, characterized by an average annual precipitation of 230 mm and reference evapotranspiration of about 2100 mm yr-1. Soil is sandy loam (clay = 8%, silt = 31% and sand = 61%) with a bulk density equal to about 1.60 g cm-3 for the layer 0-80 cm. Potato crop 'Solanum tuberosum L.', cultivar Spunta, was seeded on January 15; plants were spaced 40 cm along the rows, with distance between the rows equal to 80 cm.
Experiments were carried out in 2014 on two plots with similar management and irrigated with water, having an ECw value of 3.32 dS m -1. The crop is irrigated by a SDI system, with a single pipe per plant row. Each pipe, having a nominal diameter of16 mm and emitters spaced 40 cm apart, was installed at 20 cm depth; co-extruded emitters were characterized by a flow rate equal to 4.0 l h-1 at a nominal pressure of 100 kPa. Standard meteorological data are recorded from a weather station placed about 300 m far from the experimental area. Eight irrigation were supplied all crop cycle of potato for both treatment Time Domain Reflectometry (TDR) was used to determine the volumetric soil water content using a portable soil moisture monitoring system 'TRIME FM' probe, with a precision of about ± 0,03 cm3 cm-3, as obtained by a site-specific calibration previously carried out by Douh (2012). In each treatment, a total of three measurement tubes were installed around one emitter, the access tubes were located near the emitter, as well as at 25 cm and 50 cm from emitter, the vertical profile of soil water content was monitored during the investigation period, by measuring every 10 cm, in the layer 0-50 cm. Potato development cycle is about 120 days. At the end of the cycle, the maximum root density was observed between 35 and 45 cm depth for both treatments T1 and T2. Soil electrical conductivity in T1 and T2 was determined on saturated extract (ECe) every 15 days, using soil samples collected at three different depths, (from 0 - 20 cm, 20-40 cm and 40-60 cm) along a vertical profile near the emitter. Considering that the method is destructive, each measurement was carried out in correspondence of a different emitter. At harvesting the crop yield was determined by weighting, for both the treatments, the total production obtained in ten plants.
2.2. Model simulation description and assumptions
HYDRUS-2D, which incorporates a numerical solution of Richard’s equation (Simunek et al.2006) was applied to simulate the distribution of water and solute around a buried emitter, so to reproduce the axis symmetrical physical process occurring during the experiments. The simulation domain was assumed 80 cm depth and 40 cm width. A single emitter, characterized by a radius of 1.0 cm and placed at 20 cm below the soil surface, was considered as a punctual source of water and solutes. The domain was discretized with 837 nodes, corresponding to 1578 triangular elements, as illustrated in Figure 1. A constant flux density of 318 cm h-1, obtained by dividing the emitter flow discharge of 4.0 l h-1 by the surface of a sphere having a diameter of 20mm (slightly higher than the pipe diameter), was assumed at the emitter boundary surface during irrigation, whereas the absence of flux was considered during the redistribution processes. Even if according to Shani and al. (1996) depending on the emitter’s flow rate, on the soil texture and on the dimension of the cavity around the emitter a rising positive back pressure could occur, the assumption to suppose a constant flux density is consistent with the quite high sand content.
|
Figure 1. Boundary condition used in simulation |
2.3. Statistical analysis for model evaluation
The performance of Hydrus-2D model was evaluated by considering the Mean Bias Error (MBE), Root Mean Square Error (RMSE), and Nash-Sutcliffe model efficiency coefficient (E) (Nash and Sutcliffe, 1970; Willmott, 1980).
(1)
(2)
(3)
where Xsim,i and Xobs,i represent the simulated and observed values of the considered variable at the time i and N the number of measured data. RMSE has been largely used to evaluate the model’s performance, because of the advantage to express the error in the same units of the considered variable (Legates and McCabe, 1999), whereas MBE intends to indicate the average model bias, and therefore the average over- or under-prediction. On the other side, the Nash-Sutcliffe efficiency index (E) is normally used to assess the predictive power of the considered models. A value of the index E=1 corresponds to the perfect match between simulated and observed data, whereas, a value E = 0signifies that model predictions are as accurate as the mean of measured data. Negative values of E instead, occur when the observed mean predicts better than the considered model. In other words, the closer to 1 is the efficiency index, the more accurate the model prediction.
3. Result and Discussion
3.1. Agro-meteorological characterizations
Daily values of agro-meteorological variables (global solar radiation, Rg, relative humidity, RH, air temperature, Tair, wind speed at 2 m, V2m, rainfall, P, and reference evapotranspiration, ET0), for the period January 15(DOY 16), to May 6 (DOY 126), 2014 are shown in fig. 2. According to the climatic data, significant rainfall events occurred at DOY 32 and DOY 73, whereas less severe events were registered in the period immediately following, after which rainfall decreased notably, except that for a few events before harvesting. Reference evapotranspiration during the growing season ranged from 0.8 mm/d (DOY 15) to about 4.0 mm/d (DOY 126). Precipitation and irrigation events for both treatments T1 and T1 were showed in fig 3.
(a) |
(b) |
(c) |
(d) |
Figure 2. a-d. Daily values of a) air temperature Tair, and relative humidity, RH, b) global solar radiation, Rg, c) wind speed at 2 m above the ground, V2m, d) reference evapotranspiration, ET0, and precipitation, P, measured during the growth season 2014 (from DOY 16 to DOY 126) |
|
Figure 3. value of rain P, irrigation events for T1 and T2 during the growing season |
3.2. Comparison between simulated and measured soil water contents
The comparison between measured and simulated soil water contents at different positions around the emitter, are illustrated in figs. 4 and 5, for treatments T1 and T2 respectively. As can be observed, Hydrus 2D can reproduce the evolution of soil water content at different distances and depths from the emitters. The shift between simulated and measured soil water content, could be ascribed to a defective parameterization of the to player soil hydraulic functions, as well as to possible air gaps occurred, near the soil surface, between the access tube and the surrounding soil (Rallo and Provenzano, 2014). Moreover, for both the treatments a slight decrease of soil water content can be observed from the beginning of growth season till roughly DOY 75 (crop is at its full development stage), after which it is evident a quicker reduction, because of the relatively high potential croptranspiration. At the same time, it is noteworthy that in both treatments, during the period of stolonization and tuber building, approximately from DOY 55 to DOY 75, soil water content in the root zone is never allowed to fall below a value of about 0.22 cm3cm-3. This circumstance evidenced that during this period the crop was never subject to substantial water deficit conditions that may have had an adverse effect on crop yield. Anyway, to provide a quantitative measurement of the goodness of fit, table (1) shows the values of MBE,RMSE, evaluated for treatments T1 and T2. The three statistical indices were calculated by considering all data available as can be observed from table 1, RMSE values, equal to 0.037 cm3 cm-3 and 0.031 cm3cm-3 for treatments T1 and T2, resulted of the same order of magnitude of the error associated to the measurements (±0.03). Moreover, the values of MBE were approximately close to 0, whereas the Nash-Sutcliffe efficiency index, E, resulted positive and respectively equal to 0.24 and 0.21, for the two treatments. These results confirm the ability of Hydrus-2D model to simulate accurately the soil water content distribution around a buried emitter, even considering the patterns of root water uptake during the whole growth season. The statistical indices presented in table 3 also show that a slightly better performance of Hydrus-2D was obtained in treatment T1compared to T2. This recognized difference could be ascribed to the restrictive assumption of considering similar root distributions for both treatments. In fact, due to the different soil water status characterizing the treatments during the full development stage, it is possible that the plant could have extracted soil water by deepening or enlarging part of the root system, so involving a region outside the simulated root volume (Phogatet al, 2012).Similarly, to what observed by Skaggs et al. (2004), it can be therefore concluded that Hydrus-2D can well predict soil water contents at different distances and depths around a buried emitter even if, according to what observed by Mguidiche et al. (2014) in similar experiments, soil water status was recognized accurate only in the root zone and not locally, as currently verified.
|
|
|
|
|
|
Figure 4. Measured and simulated soil water content at 10, 30, 50 cm depth and at distances of 0 and 25 cm from the emitter for treatment T1 |
|
|
|
|
|
|
Figure 5. Measured and simulated soil water content at 10, 30, 50 cm depth and at distances of 0 and 25 cm from the emitter for treatment T2 |
Table 1. Results of the statistical comparison between simulated and measured SWCs |
||
|
T1 |
T2 |
RMSE |
0.37 |
0.031 |
MBE |
0.007 |
0.015 |
E |
0.24 |
0.21 |
3.3. Soil salinity
Fig 6 shows, for treatments T1 and T2, simulated and measured values of soil electrical conductivity determined on satured paste extract. For each simulated ECe, a certain variability of the measured value can be observed with higher value observed for treatments T2 (RMSE=0.99, MBE=-0.025) compared to T1 (RMSE=0.96, MBE=0.004). Coefficient E was negative for both treatments occur that the observed mean predicts better than the considered model. The reason for such a high variability observed in both treatments could be due to the spatial variability of ECe measured in the soil surrounding different emitters, as well as to the gradient of salt. Despite the difference between the simulated and measured values of ECe being statistically not significant, dispersion of the point around the 1:1 line suggest that the model could be acceptable for predicting the mean ECe values and to evaluate the seasonal dynamic of salt concentration around the buried emitter. A similar variability was found by Roberts et al. (2009), even if in their results a certain under estimation of the predicted ECe, compared to the measured values has been observed. According to these authors, the root growth and their distribution within the soil profile, as well as the conservative or non-conservative nature of the solute, may play an important role in simulating the seasonal dynamics of water and solutes in the soil and therefore, a better Knowledge of these variables could lead to improve the simulation results.
|
Figure 6. Simulated and measured soil electrical conductivity for T1 and T2 |
Table 2. Results of the statistical comparison between simulated and measured electrical conductivity |
||
|
T1 |
T2 |
RMSE |
0.96 |
0.99 |
MBE |
0.004 |
-0.025 |
E |
-0.29 |
-0.38 |
4. Conclusion
Drip technology under the saline environment could be quite suitable and useful solution for irrigation in saline environment. Results of this study indicate that the HYDRUS-2D model is able to simulate, with good accuracy, the mean soil water content in the root zone of potato crop and its seasonal trend. Moreover the model can be considered acceptable for predicting the salt concentration in the soil.
5. References
American Society of Agriculture and Biological Engineers (ASABE) (2007). Soil and water Terminology.PaperASAES526.3St Joseph,Mich;54pp.
Douh B.(2012). Etude théorique et expériemtale de l’irrigation goutte à goutte souterraine sur une culture de mais (Zea mays.L.).PhD Thesis, Higher Agronomic Institute of Chott Meriem (sousse),Tunisia; 210pp.
Douh B, Mguidiche, A, Bhouri Khila S, Mansour M, Harrabi R., Abdelhamid, B.(2013). Yield and Water Use Efficiency of cucumber (Cucumis sativus L.) conducted under subsurface drip irrigation system in a Mediterranean Climate,. IOSR Journal of Environmental Science, Toxicology and Food Technology (IOSR-JESTFT), 2(4), pp 46-51.
Fabeiro C, Martin de Santa Olalla F, Juan JA (2001). Yield and size of deficit irrigated potatoes. Agric. WaterManage. 48: 255- 266.
GhazouaniH, Douh M’hamdi B, Autovino, Mguidiche Bel haj A, Rallo G, Provenzano G, Boujelben A.(2015). OptimizingSubsurface Dripline Installation depth with Hydrus 2D/3D to improve irrigation water Use efficiency in the central Tunisia, Int. J. Metrol. Qual. Eng. 6, 402, 8p.
Hamdy A, Abu-Zeid M, Lacirignola C(1995). Water crisis in the Mediterranean: Agricultural demand management. Water Int. 4, 515–526.
Legates, DR., McCabe, J(1999). Evaluating the use of ‘goodness-of fit’ measures in hydrologic and hydroclimatic model validation. Water Resources Research, 35(1), 233-241, Paper number1998WR900018.
Mguidiche, A, Provenzano G, Douh B, Khila S, Rallo G, Boujelben A (2014). Assessing Hydrus-2D to simulate soil water content (SWC) and salt accumulation under a SDI system: Application to a potatoes crop in a semi arid area of central Tunisia. Published online on Irrigation and Drainage. DOI:10.1002/ird.1884
Nash JE, Sutcliffe J.V.(1970). River flow forecasting through conceptual models. Journal of Hydrology, 10(3), 282-290. DOI:10.1016/0022-1694(70)90255-6.
Phogat V, Skewes MA, Mahadevan M, Cox JW (2012). Evaluation of soil plant system response to pulsed drip irrigation of an almond tree under sustained stress conditions. Agricultural Water Management, 118(1), 1-11. DOI: 10.1016/j.agwat.2012.11.015
Porter GA, Opena, GB, Bradbury, WB, McBurnie, JC, Sisson, JA (1999). Soil management and supplemental irrigation effects on potato. I. Soil properties, tuber yield, and quality. Agronomy Journal 91, 416–425
Postel S(1996). Forging a sustainable water strategy. In: Brown, R.L. (Ed.), State of the World 1996. Earthscan Publication Ltd., London, pp. 40–59.
Rallo G, Provenzano G.,(2014). Discussion of “Laboratory and field calibration of the Diviner 2000 probe in two types of soil” by J. Haberland, PhD, R. Galvez, C. Kremer, PhD, and C. Carter. Published online on Journal of Irrigation and Drainage Engineering, ASCE. DOI: 10.1061/(ASCE)IR.1943-4744.0000687.
Roberts T, Larazovich N, Warrick AW, Thompson TL(2009). Modeling salts accumulation with subsurface drip irrigation using HYDRUS-2D. Soil Sci. Soc. Am. J., 73, 233-240.
Shani U., Xue, S.,Gording Kats., R.,Warrick, A.W.,(1996). Soil limiting flow from subsurface emitters. I: Pressure measurements. J. Irrig. Drain. Eng., 122(5), 291-295.
Simunek, J., Van Genuchten., M.T, Sejna, M.(2006). The HYDRUS 2D softawre Package for simulating tow and Three Dimensional Movement of water, Heat and Multiple solutes in Variability Saturated Media .Technical manual.Version1.0PC Progress:Prague,Czech Republic;241 pp.
Skaggs T, Trout T, Šimůnek J, Shouse P(2004). Comparison of HYDRUS-2D Simulations of Drip Irrigation with Experimental Observations. Journal of Irrigation and Drainage Engineering, ASCE.130(4), 304–310. doi: 10.1061/(ASCE) 0733-9437.
Willmott CJ(1980). On the validation of models. Physical geography 2(2), 184-194. DOI:10.1080/02723646.1981.10642213.