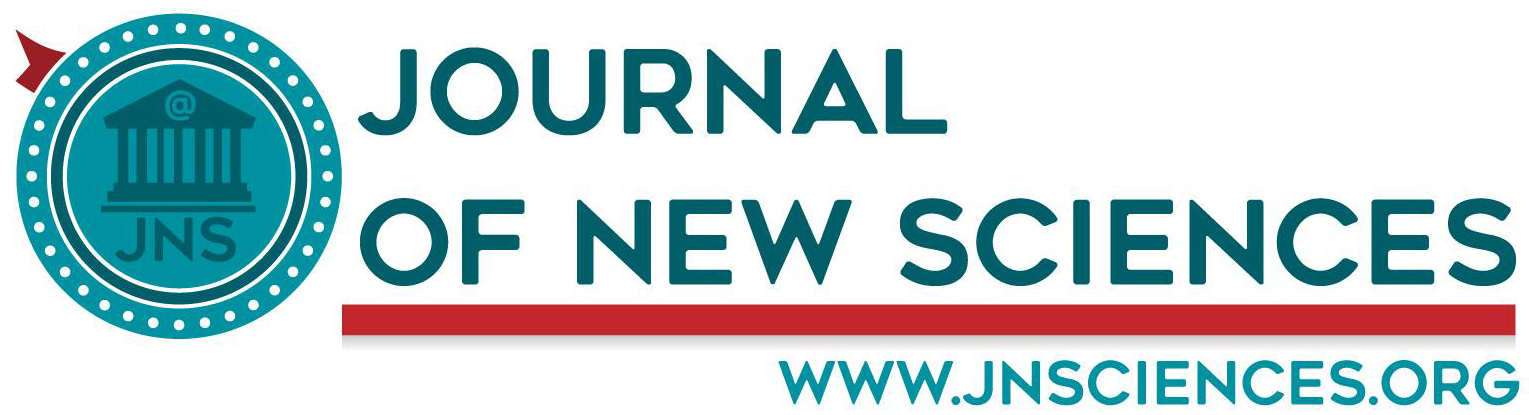
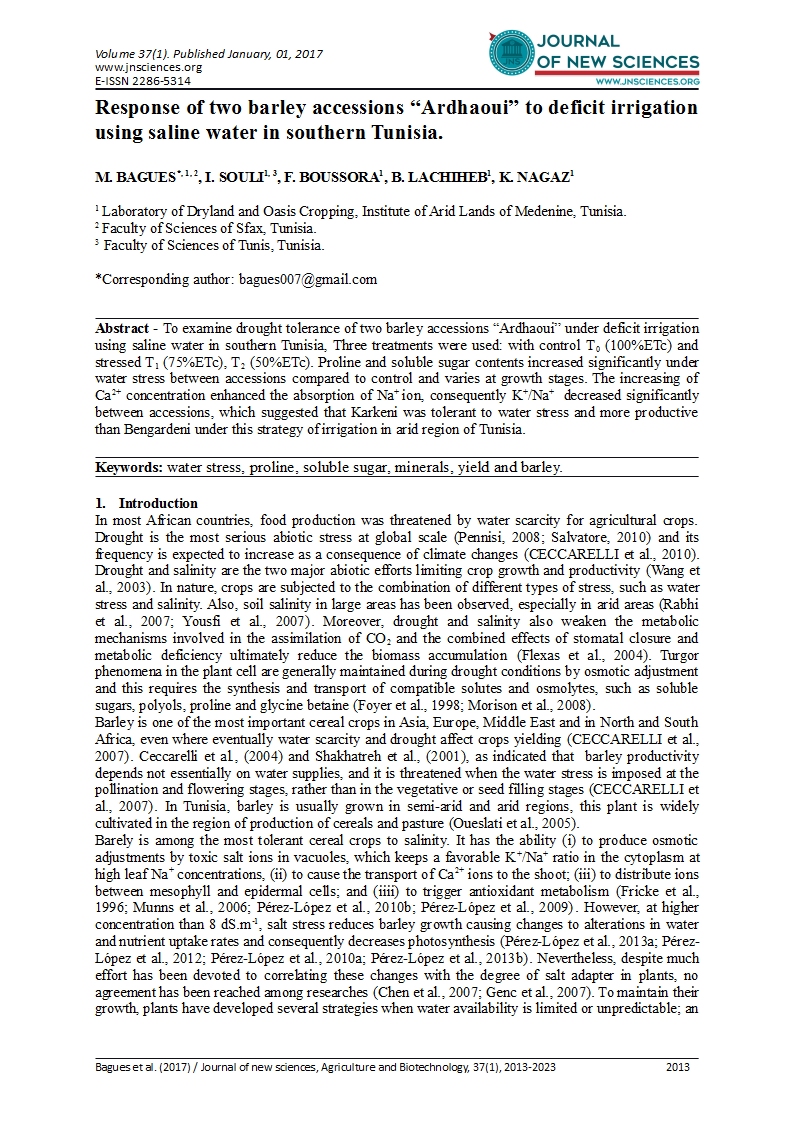
- Category: Volume 37
- Hits: 12347
Response of two barley accessions “Ardhaoui” to deficit irrigation using saline water in southern Tunisia
M. Bagues*, 1, 2
I. Souli1, 3
F. Boussora1
B. Lachiheb1
K. Nagaz1
1 Laboratory of Dryland and Oasis Cropping, Institute of Arid Lands of Medenine, Tunisia.
2 Faculty of Sciences of Sfax, Tunisia.
3 Faculty of Sciences of Tunis, Tunisia.
Abstract - To examine drought tolerance of two barley accessions “Ardhaoui” under deficit irrigation using saline water in southern Tunisia, Three treatments were used: with control T0 (100%ETc) and stressed T1 (75%ETc), T2 (50%ETc). Proline and soluble sugar contents increased significantly under water stress between accessions compared to control and varies at growth stages. The increasing of Ca2+ concentration enhanced the absorption of Na+ ion, consequently K+/Na+ decreased significantly between accessions, which suggested that Karkeni was tolerant to water stress and more productive than Bengardeni under this strategy of irrigation in arid region of Tunisia.
Keywords: water stress, proline, soluble sugar, minerals, yield and barley.
-
Introduction
In most African countries, food production was threatened by water scarcity for agricultural crops. Drought is the most serious abiotic stress at global scale (Pennisi, 2008; Salvatore, 2010) and its frequency is expected to increase as a consequence of climate changes (CECCARELLI et al., 2010). Drought and salinity are the two major abiotic efforts limiting crop growth and productivity (Wang et al., 2003). In nature, crops are subjected to the combination of different types of stress, such as water stress and salinity. Also, soil salinity in large areas has been observed, especially in arid areas (Rabhi et al., 2007; Yousfi et al., 2007). Moreover, drought and salinity also weaken the metabolic mechanisms involved in the assimilation of CO2 and the combined effects of stomatal closure and metabolic deficiency ultimately reduce the biomass accumulation (Flexas et al., 2004). Turgor phenomena in the plant cell are generally maintained during drought conditions by osmotic adjustment and this requires the synthesis and transport of compatible solutes and osmolytes, such as soluble sugars, polyols, proline and glycine betaine (Foyer et al., 1998; Morison et al., 2008).
Barley is one of the most important cereal crops in Asia, Europe, Middle East and in North and South Africa, even where eventually water scarcity and drought affect crops yielding (CECCARELLI et al., 2007). Ceccarelli et al., (2004) and Shakhatreh et al., (2001), as indicated that barley productivity depends not essentially on water supplies, and it is threatened when the water stress is imposed at the pollination and flowering stages, rather than in the vegetative or seed filling stages (CECCARELLI et al., 2007). In Tunisia, barley is usually grown in semi-arid and arid regions, this plant is widely cultivated in the region of production of cereals and pasture (Oueslati et al., 2005).
Barely is among the most tolerant cereal crops to salinity. It has the ability (i) to produce osmotic adjustments by toxic salt ions in vacuoles, which keeps a favorable K+/Na+ ratio in the cytoplasm at high leaf Na+ concentrations, (ii) to cause the transport of Ca2+ ions to the shoot; (iii) to distribute ions between mesophyll and epidermal cells; and (iiii) to trigger antioxidant metabolism (Fricke et al., 1996; Munns et al., 2006; Pérez-López et al., 2010b; Pérez-López et al., 2009). However, at higher concentration than 8 dS.m-1, salt stress reduces barley growth causing changes to alterations in water and nutrient uptake rates and consequently decreases photosynthesis (Pérez-López et al., 2013a; Pérez-López et al., 2012; Pérez-López et al., 2010a; Pérez-López et al., 2013b). Nevertheless, despite much effort has been devoted to correlating these changes with the degree of salt adapter in plants, no agreement has been reached among researches (Chen et al., 2007; Genc et al., 2007). To maintain their growth, plants have developed several strategies when water availability is limited or unpredictable; an adaptation to the lack of water by improving their absorption, accumulation of compatible solutes and mineral nutrients during to maintain turgor, metabolic activity and absorption of water in case of fall soil water potential (Hummel et al., 2010). Moreover, synthesis of osmo-regulators such as soluble sugars and proline in the event of severe stresses (Ashraf & Foolad, 2007; Delauney & Verma, 1993).
To select adapted plant to Mediterranean-type climates, it is mandatory to study the phenological, morphological, physiological and agronomic parameters (González & Ayerbe, 2010; González et al., 1999) in order to improve plant adaptation to drought. Barley is one of suitable and profitable crops in these conditions (González et al., 2007).
The objectives of this work is (i) to evaluate the biochemical and agronomic performances of two barley accessions subjected to deficit irrigation at different growth stages in dryland, (ii) to assess the responses of this material to water stress as tool of selection and (iii) to determine the irrigation strategy to improve water productivity of barley in southern Tunisia.
-
Materials and Methods
-
Experimental conditions
-
The experiment was conducted at the experimental site of the Institute of Arid Lands of Medenine (IRA: 33°29’3’’N, 10º38’46’’E, Altitude 184m in the South-East of Tunisia). The climate is Mediterranean, with hot, dry summers and mild winters an average annual rainfall of 125 mm. Minimum temperatures recorded during the months of November to May, are included between 3.5 and 15.7 °C, while maximum temperatures are between 16 and 39.8 °C in the same period.
|
Figure 1: Decadal average temperatures and rainfall in the region of Elfjè, Medenine (source: Weather Station of the Institute of Arid Regions of Medenine 2014)
|
-
Plant material
Two barley accessions cv. Ardhaoui (six rows) were used in this study. Seeds were collected from two different regions in southern Tunisia (“karkeni” from Karkenah and “Bengardeni” from Bengardene) to compare their behavior when grown under conditions of water stress.
-
Experimental design
The experimental plan consisted of 3 blocks with an area of 352 m2. Each block (117.3 m2) was divided into three equal plots of 39.1 m2 (T0 (100%ETc), T1 (75%ETc) and T2 (50%ETc)) irrigation treatments, each treatment was divided into two sub-blot contain the two accessions Karkeni and Bengardeni, each sub-blot contains 6 rows spaced 40 cm (in total there will be 108 lines) (Figure 2), the distance between the emitters is of 16 cm. Before sowing, 175kg.ha-1 N, 100kg.ha-1 P and 125kg.ha-1 K were equally distributed for all rows. The seeds were sown on depth of 2 to 3 cm, the planting density is 230 plants per m2. All blocks were drip irrigated with water from a well having an ECi of 10.8 dS.m-1. Each dripper had a 2 l/h flow rate. A control mini-valve in the lateral permits use or non-use of the dripper line.
44m
Block 3 =14.6m Block 2 =14.6m Block 1 = 14.6m
|
||||||||||||||||||||||||||||||||||||||||||||
Figure 2: Experimental design : K (Karkeni), B (Bengardeni), T0 (100%ETc full irrigation), T1 (75%ETc deficit irrigation) and T2 (50%ETc deficit irrigation)
|
-
Measurement
-
Proline and soluble sugars determination
-
The technique used is that of Troll and Lindsley (1955). The quantification of the reaction by ninhydrin proline spectrophotometric measurement. Reacting is characterized by the appearance of the red color due to the proline-ninhydrin complex. Of each accession and each treatment, 100 mg of fresh material are removed from the leaves of the flourished 3rd row from the top. These samples were cut into small pieces and placed in test tubes containing screwed 2 ml Methanol (40%) and heated to boiling in a water bath at 85°C for 60 min. After cooling samples, 1 ml of the extract of each was removed and placed into new tubes to which was added 1 ml of acetic acid and 1 ml of a solution containing 120 ml H2O, 300 ml acetic acid, 80 ml ortho-phosphoric acid "H3 PO4, density 1.7" and 25 mg of ninhydrin. The mixture is heated to boiling for 30 min. The solution turns to red. After cooling, was added 5 ml of toluene in each tube with stirring for two phases, then removed the upper phase in which a pinch of Na2SO4 was added to removing water and the optical density was measured by a spectrophotometer thereafter (Bio-Rad Smart SpecTM 3000) at the wavelength of 528 nm.
The content of soluble sugars, 100 mg of fresh material is taken to which is added 5 ml of 80% methanol and the samples were heated in a water bath at 70°C for 30 minutes. The soluble sugar content was determined by the phenol-sulfuric acid method (Roby and White, 1987). 1 ml is removed from the extract which was added 1 ml of phenol 5% and 5 ml of concentrated sulfuric acid. After stirring and cooling, determine the absorbance in a spectrophotometer at 640 nm. The calibration is performed by glucose solutions with concentrations of 0.05 to 0.3 mg.ml-1.
Concentration of soluble sugars (μmol/mg MF) = α * Abs * 1 / Re
With α = slope of the calibration curve, Re = m / v = 0.1 / 5, Abs = read.
-
Yield and yield components
The measured yield components agronomic were: Plant height (PH), dry matter (DM), spike length (SL), tillers number per m2 (TN), spikes number per m2 (SN), grain number per spike (GN), 1000 grains weight (TWG) and grain yield (GY).
Table1: Unit, time of measurement, replication and protocol of yield and yield components.
|
||||
Parameters |
Unit |
Time of measurement |
Replication number |
Protocol |
PH |
cm |
In every growth stage |
7 |
A simple measure |
DM |
g |
At maturity |
7 |
A simple measure |
SL |
cm |
At maturity |
7 |
A simple measure |
TN |
number |
At maturity |
7 |
simple manual counting |
SN |
number |
At maturity |
7 |
simple manual counting |
GN |
number |
At maturity |
7 |
simple manual counting |
TWG |
g |
At maturity |
7 |
With automatic counter grains CONTADOR |
GY |
q/ha |
At maturity |
7 |
GY=TWG*SN*GN
|
-
Minerals analysis
Samples were totally dried at 100±5°C to constant weight. Then, 1g of each dry sample was incinerated during 4 h at 550°C. Ashes were mixed with 4 ml of ultra-pure water and 1 ml of concentrated Hcl. The solution was heated until boiling, then filtered and adjusted to 100 ml with ultra-pure water. This solution will be used for mineral analysis. All minerals contents were determined with an Atomic Absorption Spectrometry (Thermo SCIENTIFIC iCE 3000 AA Spectrometer). The contents of sodium (Na+), potassium (K+) and calcium (Ca2+) in the dry matter were calculated as:
C (mg/100g) = c (mg/l)* 10-3 *50*100*DF
Where C is the concentration of mineral (mg/100 g DM), “c” is the concentration of mineral (mg/l), DF is the factor of dilution.
-
Statistical analysis
All data presented were mean values of each treatments and were taken on three (sugars, proline and minerals) and seven (yield and yield components) replicates. Analyses of variance (ANOVA: General Linear Model) was carried out using the statistical package SPSS v 20. Relationships between yield and yield components were determined using Pearson’s simple correlation test (Pearson).
-
Results
-
Soil salinity and humidity
-
The final average ECi values (0-60 cm soil depth) under different treatments was presented in Figure 3. Initial soil salinity determined at the time of planting was 11.23 dS.m-1. However, soil salinity decrease in deficit irrigation treatments (T1 and T2) than full irrigation (T0). ECi values were in a decreasing order 50%ETc > 75%ETc > 100%ETc, also, soil salinity varies between growth stages due to the sourcing of rainfall (24, 48 and 49 mm) (Figure 1) which causes increased soil moisture and consequently decreased salinity.
The values of ECi varies between growth stages, soil salinity has the highest values at tillering and booting stages, this is because of excessive irrigation. At maturation stage, soil salinity decreases with decreasing irrigation.
|
Figure 3: Changes in soil salinity and humidity according to growth stages and irrigation treatments
|
-
Determination of proline content
Analysis of variance showed significant difference between the irrigation treatments at p<0.01. Water stress increase significantly proline content for both T1 (75%ETc), T2 (50%ETc) treatments compared to control T0 (100%ETc) for the two accessions (Table 2). Furthermore, Tillering stage present the high proline levels which increase from 0.085 to 0.1 mg.g-1 for “Karkeni” and from 0.063 to 0.091 mg.g-1 for “Bengardeni” respectively for T0 and T2 treatments (Figure 4).
|
Figure 4: variation of proline content in the tow barley accessions with the irrigation treatments during the growth stages |
For all treatments and during the growth stages the difference between accessions showed a highly significant at (p<0.001). The interaction between treatments, accessions and growth stages (T*A, T*GS, A*GS and T*A*GS) showed also a highly significant differences at (p<0.001) (Table2).
-
Determination of soluble sugar content
Soluble sugar content increase significantly between the irrigation treatments for the tow barley accessions (p<0.01). In fact, in the Tillering stage, the soluble sugar content increase by 16% and by 19% respectively for “Karkeni” and “Bengardeni” for the treatments T1 (75%ETc) and T2 (50%ETc) (Figure 5).
The difference between accessions is not significant (Table 2). Moreover, at Booting and Heading stages the difference between accessions is significantly at (p<0.01). However, at the Booting stage, soluble sugar content reduce significantly (p<0.01) for the Karkeni compared to Bengardeni. Our results showed a highly significant (p<0.001) between growth stages. Their interaction T*A no significant, GS*A, GS*T and T*A*GS showed a significant difference at (p<0.1, p<0.001 and p<0.01) respectively.
|
|||
Figure 5: variation of soluble sugar content in the tow barley accessions with the irrigation treatments during the growth stages |
|||
Table 2: Effect of accessions, treatments and growth stages on soluble sugar and proline and their interaction (T*A, GS*A, T*GS and T*GS*A)
|
|||
ANOVA |
ddl |
Soluble sugar |
Proline |
Treatment (T) |
2 |
* |
** |
Accession (A) |
1 |
ns |
*** |
Growth Stages (GS) |
2 |
*** |
*** |
T*A |
2 |
ns |
*** |
GS*A |
2 |
* |
*** |
GS*T |
2 |
*** |
*** |
T*GS*A |
4 |
* |
*** |
*, **, *** significant at p<0.05; p<0.01, p<0.001 respectively and ns: non significant |
-
Minerals composition
Water stress (T1 and T2) and growth stages caused a highly significant (p<0.001) increases in Na+ and Ca2+ contents (from 2182.65 to 2529.3 mg/100g DW) for Karkeni and (from 8.77 to 11.57 mg/100g DW) for Bengardeni respectively compared and affected significantly K+/Na+ selectivity (p<0.001).
Table 3: Effect of the treatments, accessions and growth stages on mineral composition and their interactions (T*A, A*GS, T*GS and T*GS*A)
|
||||
ANOVA |
Ca2+ |
K+ |
Na+ |
K+/Na+ |
Treatment (T) |
*** |
*** |
*** |
*** |
Accession (A) |
** |
ns |
** |
** |
Growth Stages (GS) |
*** |
*** |
*** |
*** |
T*A |
ns |
ns |
ns |
ns |
A*GS |
*** |
** |
*** |
* |
T*GS |
*** |
ns |
ns |
ns |
T*GS*A |
ns |
ns |
ns |
ns |
*, **, *** significant at p<0.05; p<0.01 and p<0.001 respectively; ns: non-significant
|
|
Figure 6: Ca2+, K+ and Na+ contents and K+/Na+ selectivity under irrigation treatments (T0 100%ETc, T1 75%ETc and T2 50%ETc) during the growth stages.
|
For all growth stages and under water stress, K+ content decrease significantly at p<0.001 for the tow accessions. In fact, at Tillering stage, K+ content decreased from 2838.79 to 2339.79 mg/100g DW in Karkeni and from 2703.46 to 2601.52 in Bengardeni. With water stress T1 and T2 K+/Na+ ratio decreased (from 1.17 to 0.92 in Karkeni and from 1.4 to 1.25 mg/100g DW in Bengardeni) in the same stage (Figure 6d). At Booting stage, Bengardeni represented the high levels compared to Karkeni for the Na+ contents.
-
Yield and yield components
To analyze the effect of full and deficit irrigation treatments on the final yield, eight parameters were retained: plant height, tiller number, spike length, total dry matter, grain yield, spike number / m2, seed number / spike and 1000 grains weight. The data are showed in Figures 7a, 7b, 7c, 7d, 7e, 7f, 7g and 7h.
Plant height was significantly in the T1 and T2 than T0 treatments (Figure 7a) also between accessions p<0.001. However, dry matter production was not significantly affected by irrigation treatments, a highly significant difference between the accessions (p<0.001). Therefore, water stress caused a significantly reduction in the tiller number and a subsequently reduction on the number of spike per plant.
Spike length, grains number per spike and 1000 grains weight decreased significantly between the two accessions in the T1 and T2 treatments compared to T0. Consequently, grain yield showed a highly significant between all irrigation treatments p<0.001 (Figure 7b, 7d, 7f and 7h; Table 2).
|
Figure 7: yield and yield components in the tow barley accessions under irrigation treatments (T0 100%ETc, T1 75%ETc and T2 50%ETc).
|
Furthermore, we noticed that grain yield was positively correlated to spike length (r= 0.784**), grain number per spike (r= 0.836**) and 1000 grains weight (r= 0.881**) at p<0.01 (Table 4).
Table 4: Effect of the treatments, accession on yield and yield components and their interaction (T*A).
|
|||||||||
ANOVA |
ddl |
PH |
TN |
SN |
SL |
DM |
TWG |
GS |
GY |
Treatment |
2 |
*** |
** |
*** |
*** |
ns |
*** |
*** |
*** |
Accession |
1 |
*** |
ns |
* |
** |
*** |
*** |
*** |
*** |
T*A |
2 |
* |
ns |
ns |
ns |
ns |
*** |
ns |
ns |
*, **, *** significant at p<0.05; p<0.01 and p<0.001 respectively; ns: non-significant
|
In fact, a highly significant decrease on plant height, dry matter, grains per spike and 1000 grains weight in the two barley accessions. A significant positive correlation observed between tiller number (r= 0.561**), number of spikes per plant (r= 0.960**), spike length (r= 0.784**), number of grain per spike (r= 0.881**) and grain yield.
Table 5: Correlation analysis of yield components with the grain yield GY (q/ha).
|
|||||||
|
PH |
TN |
SN |
SL |
DM |
TWG |
GS |
GY |
0.800** |
0.561** |
0.960** |
0.784** |
0.427** |
0.881** |
0.836** |
*, ** significantly at p<0.05 and p<0.01 respectively.
|
-
Discussion
In our study, in addition to water deficit, the experiment was conducted in field and in saline conditions (initial soil salinity ECi = 11.23 dS.m-1, salinity of irrigation water ECi = 10.8 dS.m-1), so the plants that grow on this type of soils are often subjected to salinity (Abbas et al., 2015; Biswas & Biswas, 2014). Indeed, results show that the water deficit responses triggers biochemical and agronomic changes on barley plants and at the same time promotes adaptations mechanisms to ensure the survival and productivity of plants in serious conditions (Bartels & Sunkar, 2005). However, ECi values increases under drought (T1 and T2) than control T0 in all growth stages in an order 50%ETc > 75%ETc > 100%ETc, this increasing it may be attributed to little leaching of the soil expected under drought (Schoups et al., 2005). Accumulation of osmo-regulators such as proline and soluble sugars facilate osmo-regulation under drought and salinity has been observed in plants (Trovato et al., 2008). In present study, proline and soluble sugars increases under water stress (T1 and T2) and varies between accessions during the growth stages, this augmentation enable plants to tolerate drought (Kocsy et al., 2005). Proline content present the higher level at Tillering than Booting and Heading stages; he is from 0.1 to 0.049 for Karkeni respectively at Tillering and Booting stages and from 0.91 to 0.04 for Bengardeni in the same stages ant treatments (T0 to T2) (50%) (Figure 4). This difference for this trait probably results from a different genetic potential among the varieties studied, which is widely observed also (Shao et al., 2006a; Geravandi et al., 2011). Soluble sugar increased in the same way as the proline. This variation can be explained by rainfall (Figure 3) and by physiological mechanisms of plants, (Szira et al., 2008). Highly correlation between proline and soluble sugar are observed. Similar results for (Morant-Manceau et al., 2004) and (Navarro and Rubio, 2006).
Water stress increase significantly Ca2+ content between barley accessions and irrigation treatments, it may be assumed that maintaining higher translocation of Ca2+ is an important way to reduce water stress or beneficial to improve plant tolerance to drought. Increase in Na+ ion and decrease in K+ ion content uptake interrupted ionic balance as observed in the tow accessions under all treatments (Ahmed et al., 2013; Qiu et al., 2011).
The accumulation of Na+ ion was the expense of the K+ absorption (r= -0.013), K+/Na+ ratio decreased significantly (p<0.01) between barley accessions, it is more important in Bengardeni accession than Karkeni under water treatments. The varieties used accumulate more K+ than Na+, other works found that under saline conditions the plant accumulate more Na+ than K+ MP. Lindsay et al., (2004) on durum wheat and Munns et al., (2006) on wheat and barley. The high K+/Na+ ratio indicate that the Karkeni accession is less susceptible than Bengardeni to water stress under saline conditions. These results are similar find that by (Ahmed et al., 2013; Wu et al., 2011) working on Tibetan wild and cultivated barley.
In addition, water stress showed a highly significant difference between barley accessions in yield and yield components (p<0.001).
-
Conclusion
Karkeni is less sensitive to water stress than Bengardeni, as shown by their relatively high proline and soluble sugars contents. Moreover, drought tolerance of Karkeni was found to be associated with a lower selectivity K+/Na+ ratio. Karkeni is the less sensitive to water stress and the most productive than Bengardeni under three water regimes. In addition, it can be concluded that the full irrigation (T0 100%ETc) and (T1 75%ETc) strategies offer significant advantage for yield and reduce the build-up of salinity compared to the (T2 50%ETc) irrigation practices in barley production under arid conditions.
Acknowledgement
We are very grateful for all staff of “Laboratory of Dryland an Oasis Cropping, Institue of Arid Lands of Medenine, Tunisia”, especially Tebra TRIKI and Leila Ben Yahia two laboratory technicians for all help during experiments.
-
References
Abbas G, Saqib M, Akhtar J, Haq M.A.u (2015) Interactive effects of salinity and iron deficiency on different rice genotypes. Journal of Plant Nutrition and Soil Science, 178(2), 306-311.
Ahmed I.M, Dai H, Zheng W, Cao F, Zhang G, Sun D, Wu F (2013) Genotypic differences in physiological characteristics in the tolerance to drought and salinity combined stress between Tibetan wild and cultivated barley. Plant Physiology and Biochemistry, 63, 49-60.
Morant-Manceau A, Pradier E, Tremblin G (2004) Osmotic adjustment, gas exchanges and chlorophyll fluorescence of a hexaploid triticale and its parental species under salt stress. J. Plant Physiol. 161. 25–33.
Navarro A.R, Rubio F (2006) High-affinity potassium and sodium transport systems in plants Journal of Experimental Botany; 57(5):1149-1160
Ashraf M, Foolad M.R (2007) Roles of glycine betaine and proline in improving plant abiotic stress resistance. Environmental and Experimental Botany, 59(2), 206-216.
Bartels D, Sunkar R (2005) Drought and salt tolerance in plants. Critical Reviews in Plant Sciences, 24(1), 23-58.
Biswas A, Biswas A (2014) Comprehensive Approaches in Rehabilitating Salt Affected Soils: A Review on Indian Perspective. Open Transactions On Geosciences, Scientific Online Publishing, 1(1).
Ceccarelli S, Grando S, Baum M (2007) PARTICIPATORY PLANT BREEDING IN WATER-LIMITED ENVIRONMENTS. Experimental Agriculture, 43(04), 411-435.
Ceccarelli S, Grando S, Baum M, Udupa S.M (2004) Breeding for Drought Resistance in a Changing Climate. in: Challenges and Strategies of Dryland Agriculture, (Eds.) S.C. Rao, J. Ryan, Crop Science Society of America and American Society of Agronomy. Madison, WI, pp. 167-190.
Ceccarelli S, Grando S, Maatougui M, Michael M, Slash M, Haghparast R, Rahmanian M, Taheri A, Al-Yassin A, Benbelkacem A, Labdi M, Mimoun H, Nachit M (2010) Plant breeding and climate changes. The Journal of Agricultural Science, 148(06), 627-637.
Chen Z, Zhou M, Newman I.A, Mendham N.J, Zhang G, Shabala S (2007) Potassium and sodium relations in salinised barley tissues as a basis of differential salt tolerance. Functional Plant Biology, 34(2), 150-162.
Delauney A.J, Verma D.P.S (1993) Proline biosynthesis and osmoregulation in plants. The Plant Journal, 4(2), 215-223.
Flexas J, Bota, J Loreto, F Cornic, G Sharkey T.D (2004) Diffusive and metabolic limitations to photosynthesis under drought and salinity in C(3) plants. Plant Biol (Stuttg), 6(3), 269-79.
Foyer C.H, Valadier M.H, Migge A, Becker T.W (1998) Drought-induced effects on nitrate reductase activity and mRNA and on the coordination of nitrogen and carbon metabolism in maize leaves. Plant Physiology, 117(1), 283-292.
Fricke W, Leigh R.A, Tomos A.D (1996) The intercellular distribution of vacuolar solutes in the epidermis and mesophyll of barley leaves changes in response to NaCl. Journal of Experimental Botany, 47(9), 1413-1426.
Genc Y, McDonald G.K, Tester M (2007) Reassessment of tissue Na+ concentration as a criterion for salinity tolerance in bread wheat. Plant, Cell & Environment, 30(11), 1486-1498.
Geravandi M, Farshadfar E, Kahrizi D (2011) Evaluation of some physiological traits as indicators of drought tolerance in bread wheat genotypes, Russian Journal of Plant Physiology 58, pp. 69-75.
González A, Ayerbe L (2010) Effect of terminal water stress on leaf epicuticular wax load, residual transpiration and grain yield in barley. Euphytica, 172(3), 341-349.
González A, Martín I, Ayerbe L (2007) Response of barley genotypes to terminal soil moisture stress: phenology, growth, and yield. Australian Journal of Agricultural Research, 58(1), 29-37.
González A, Martı́n I, Ayerbe L (1999) Barley yield in water-stress conditions.: The influence of precocity, osmotic adjustment and stomatal conductance. Field Crops Research, 62(1), 23-34.
Hummel I, Pantin F, Sulpice R, Piques M, Rolland G, Dauzat M, Christophe A, Pervent M, Bouteille M, Stitt M, Gibon Y, Muller B (2010) Arabidopsis plants acclimate to water deficit at low cost through changes of carbon usage: an integrated perspective using growth, metabolite, enzyme, and gene expression analysis. Plant Physiol, 154(1), 357-72.
Kocsy G, Laurie R, Szalai G, Szilágyi V, Simon-Sarkadi L, Galiba G, De Ronde J.A (2005) Genetic manipulation of proline levels affects antioxidants in soybean subjected to simultaneous drought and heat stresses. Physiologia Plantarum, 124(2), 227-235.
Morison J.I.L, Baker N.R, Mullineaux P.M, Davies W.J (2008) Improving water use in crop production. Philosophical Transactions of the Royal Society B: Biological Sciences, 363(1491), 639-658.
MP. Lindsay, E. Lagudah, and R. Munns (2004) A locus for sodium exclusion (Nax1), a trait for salt tolerance, mapped in durum wheat. Functional Plant Biology 31:1105–1114.
Munns R, James R.A, Läuchli A (2006) Approaches to increasing the salt tolerance of wheat and other cereals. Journal of Experimental Botany, 57(5), 1025-1043.
Oueslati O, Ben-Hammouda M, Ghorbal M.H, Guezzah M, Kremer R.J (2005) Barley Autotoxicity as Influenced by Varietal and Seasonal Variation. Journal of Agronomy and Crop Science, 191(4), 249-254.
Pennisi E (2008) Plant genetics. The blue revolution, drop by drop, gene by gene. Science, 320(5873), 171-3.
Pérez-López U, Miranda-Apodaca J, Mena-Petite A, Muñoz-Rueda A (2013a) Barley Growth and Its Underlying Components are Affected by Elevated CO2 and Salt Concentration. Journal of Plant Growth Regulation, 32(4), 732-744.
Pérez-López U, Robredo A, Lacuesta M, Mena-Petite A, Muñoz-Rueda A (2012) Elevated CO2 reduces stomatal and metabolic limitations on photosynthesis caused by salinity in Hordeum vulgare. Photosynthesis Research, 111(3), 269-283.
Pérez-López U, Robredo A, Lacuesta M, Muñoz-Rueda A, Mena-Petite A (2010a) Atmospheric CO2 concentration influences the contributions of osmolyte accumulation and cell wall elasticity to salt tolerance in barley cultivars. Journal of Plant Physiology, 167(1), 15-22.
Pérez-López U, Robredo A, Lacuesta M, Sgherri C, Mena-Petite A, Navari-Izzo F, Muñoz-Rueda A (2010b) Lipoic acid and redox status in barley plants subjected to salinity and elevated CO2. Physiologia Plantarum, 139(3), 256-268.
Pérez-López U, Robredo A, Lacuesta M, Sgherri C, Muñoz-Rueda A, Navari-Izzo F, Mena-Petite A (2009) The oxidative stress caused by salinity in two barley cultivars is mitigated by elevated CO2. Physiologia Plantarum, 135(1), 29-42.
Pérez-López U, Robredo A, Miranda-Apodaca J, Lacuesta M, Muñoz-Rueda A, Mena-Petite A (2013b) Carbon dioxide enrichment moderates salinity-induced effects on nitrogen acquisition and assimilation and their impact on growth in barley plants. Environmental and Experimental Botany, 87, 148-158.
Qiu L, Wu D, Ali S, Cai S, Dai F, Jin X, Wu F, Zhang G (2011) Evaluation of salinity tolerance and analysis of allelic function of HvHKT1 and HvHKT2 in Tibetan wild barley. Theoretical and Applied Genetics, 122(4), 695-703.
Rabhi M, Barhoumi Z, Ksouri R, Abdelly C, Gharsalli M (2007) Interactive effects of salinity and iron deficiency in Medicago ciliaris. Comptes Rendus Biologies, 330(11), 779-788.
Salvatore C (2010) Drought and Drought Resistance. in: Encyclopedia of Biotechnology in Agriculture and Food, Vol. null, Taylor & Francis, pp. 205-207.
Schoups G, Hopmans J.W, Young C.A, Vrugt J.A, Wallender W.W, Tanji K.K, Panday S (2005) Sustainability of irrigated agriculture in the San Joaquin Valley, California. Proceedings of the National Academy of Sciences, 102(43), 15352-15356.
Shakhatreh Y, Kafawin O, Ceccarelli S, Saoub H (2001) Selection of Barley Lines for Drought Tolerance in Low-Rainfall Areas. Journal of Agronomy and Crop Science, 186(2), 119-127.
Shao H-B, Chen X.Y, Chu L.Y, Zhao X-N, Wu G, Y, Zhao C.X, Hu Z M (2006a) “Investigation on the relationship of proline with wheat anti-drought under soil water deficits”, Biointerfaces 53, pp. 113-119.
Szira F, Bálint A.F, Börner A, Galiba G (2008) Evaluation of Drought-Related Traits and Screening Methods at Different Developmental Stages in Spring Barley. Journal of Agronomy and Crop Science, 194(5), 334-342.
Troll W, Lindsley J (1955) A PHOTOMETRIC METHOD FOR THE DETERMINATION OF PROLINE. Journal of Biological Chemistry, 215(2), 655-660.
Trovato M, Mattioli R, Costantino P (2008) Multiple roles of proline in plant stress tolerance and development. RENDICONTI LINCEI, 19(4), 325-346.
Wang W, Vinocur B, Altman A (2003) Plant responses to drought, salinity and extreme temperatures: towards genetic engineering for stress tolerance. Planta, 218(1), 1-14.
Wu D, Qiu L, Xu L, Ye L, Chen M, Sun D, Chen Z, Zhang H, Jin X, Dai F, Zhang G (2011) Genetic Variation of <italic>HvCBF</italic> Genes and Their Association with Salinity Tolerance in Tibetan Annual Wild Barley. PLoS ONE, 6(7), e22938.
Yousfi S, Wissal M, Mahmoudi H, Abdelly C, Gharsalli, M (2007) Effect of salt on physiological responses of barley to iron deficiency. Plant Physiology and Biochemistry, 45(5), 309-314.