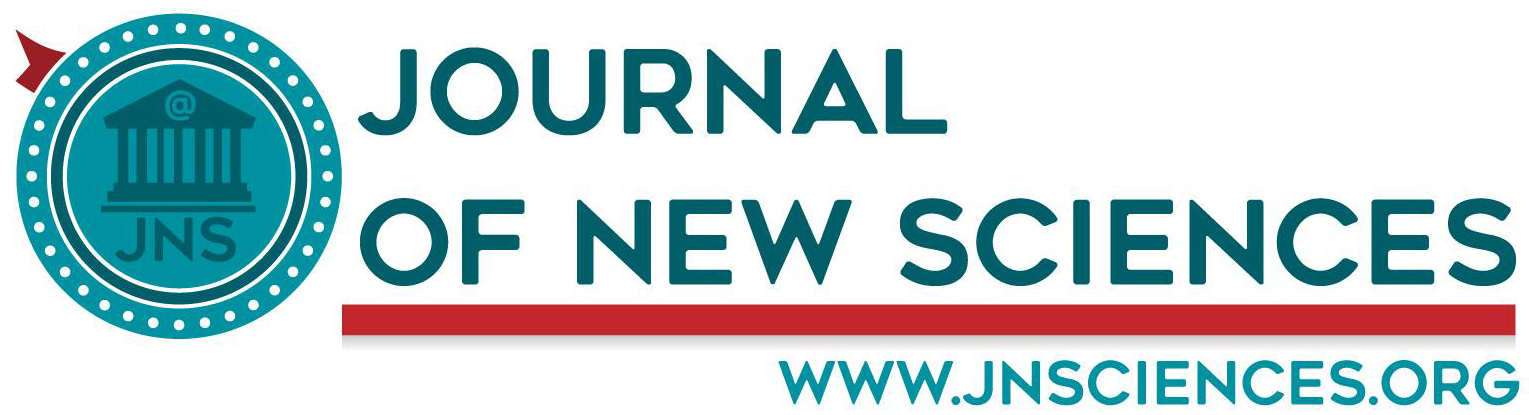
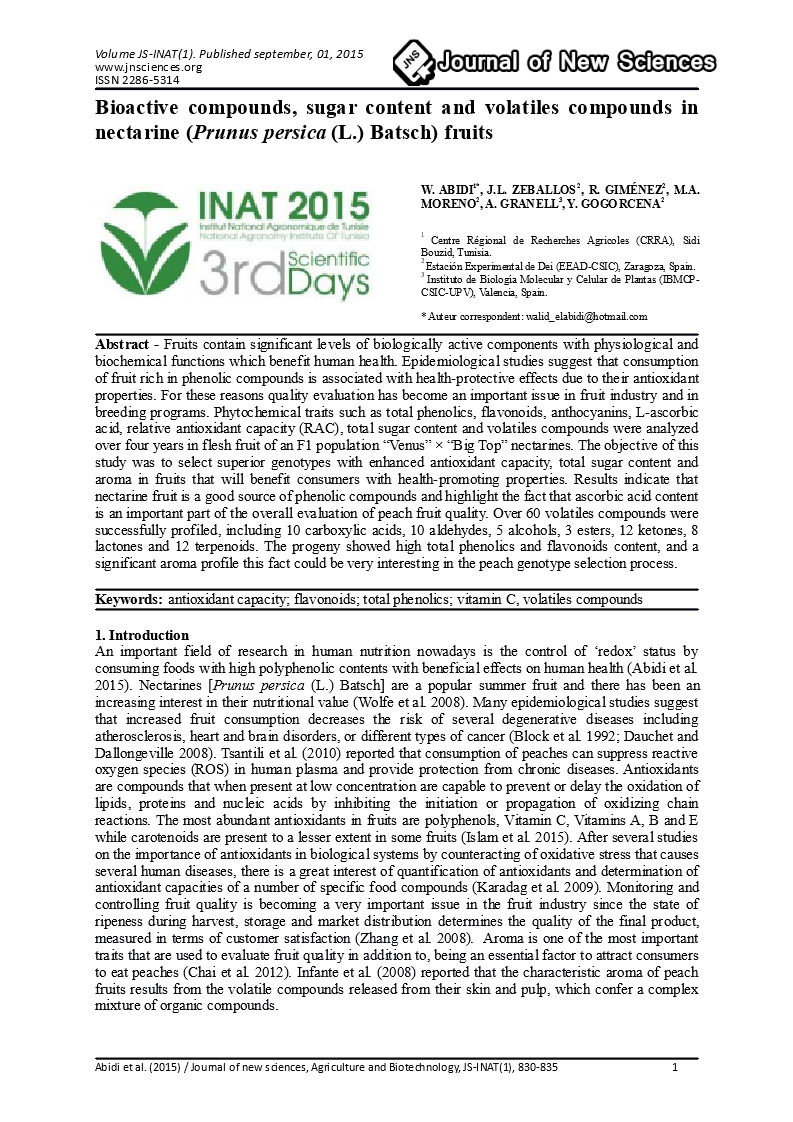
- Category: Volume Spécial (Journées Scientifiques de l'INAT)
- Hits: 5612
Bioactive compounds, sugar content and volatiles compounds in nectarine (Prunus persica (L.) Batsch) fruits
W. Abidi1*
J.L. Zeballos2
R. Giménez2
M.A. Moreno2
A. Granell3
Y. Gogorcena2
1 Centre Régional de Recherches Agricoles (CRRA), Sidi Bouzid, Tunisia.
2 Estación Experimental de Dei (EEAD-CSIC), Zaragoza, Spain.
3 Instituto de Biología Molecular y Celular de Plantas (IBMCP-CSIC-UPV), Valencia, Spain.
Abstract - Fruits contain significant levels of biologically active components with physiological and biochemical functions which benefit human health. Epidemiological studies suggest that consumption of fruit rich in phenolic compounds is associated with health-protective effects due to their antioxidant properties. For these reasons quality evaluation has become an important issue in fruit industry and in breeding programs. Phytochemical traits such as total phenolics, flavonoids, anthocyanins, L-ascorbic acid, relative antioxidant capacity (RAC), total sugar content and volatiles compounds were analyzed over four years in flesh fruit of an F1 population “Venus” × “Big Top” nectarines. The objective of this study was to select superior genotypes with enhanced antioxidant capacity, total sugar content and aroma in fruits that will benefit consumers with health-promoting properties. Results indicate that nectarine fruit is a good source of phenolic compounds and highlight the fact that ascorbic acid content is an important part of the overall evaluation of peach fruit quality. Over 60 volatiles compounds were successfully profiled, including 10 carboxylic acids, 10 aldehydes, 5 alcohols, 3 esters, 12 ketones, 8 lactones and 12 terpenoids. The progeny showed high total phenolics and flavonoids content, and a significant aroma profile this fact could be very interesting in the peach genotype selection process.
Keywords: antioxidant capacity; flavonoids; total phenolics; vitamin C, volatiles compounds
1. Introduction
An important field of research in human nutrition nowadays is the control of ‘redox’ status by consuming foods with high polyphenolic contents with beneficial effects on human health (Abidi et al. 2015). Nectarines [Prunus persica (L.) Batsch] are a popular summer fruit and there has been an increasing interest in their nutritional value (Wolfe et al. 2008). Many epidemiological studies suggest that increased fruit consumption decreases the risk of several degenerative diseases including atherosclerosis, heart and brain disorders, or different types of cancer (Block et al. 1992; Dauchet and Dallongeville 2008). Tsantili et al. (2010) reported that consumption of peaches can suppress reactive oxygen species (ROS) in human plasma and provide protection from chronic diseases. Antioxidants are compounds that when present at low concentration are capable to prevent or delay the oxidation of lipids, proteins and nucleic acids by inhibiting the initiation or propagation of oxidizing chain reactions. The most abundant antioxidants in fruits are polyphenols, Vitamin C, Vitamins A, B and E while carotenoids are present to a lesser extent in some fruits (Islam et al. 2015). After several studies on the importance of antioxidants in biological systems by counteracting of oxidative stress that causes several human diseases, there is a great interest of quantification of antioxidants and determination of antioxidant capacities of a number of specific food compounds (Karadag et al. 2009). Monitoring and controlling fruit quality is becoming a very important issue in the fruit industry since the state of ripeness during harvest, storage and market distribution determines the quality of the final product, measured in terms of customer satisfaction (Zhang et al. 2008). Aroma is one of the most important traits that are used to evaluate fruit quality in addition to, being an essential factor to attract consumers to eat peaches (Chai et al. 2012). Infante et al. (2008) reported that the characteristic aroma of peach fruits results from the volatile compounds released from their skin and pulp, which confer a complex mixture of organic compounds.
The main objective of this work were to evaluate the content of antioxidant compounds, total sugar and volatile compounds in nectarine fruits, and to study the relationships among biochemicals fruit quality traits and their health protecting properties in a nectarine segregating F1 population.
2. Material and Methods
2.1. Plant material
The progeny assayed was a segregant F1 population of 75 seedlings obtained from a controlled cross between P. persica cvs. ‘Venus’ (female parent) and ‘BigTop’ (male parent). ‘Venus’ is a FMF (freestone melting flesh) nectarine cultivar, whereas ‘BigTop’ is a CMF (clingstone melting flesh) nectarine cultivar. The segregant population is entirely melting flesh, either cling- or freestone. Progenies were established during 2001 in a plot at the Experimental Station of Aula Dei (Zaragoza, Spain).
2.2. Biochemical analyses
Twenty representative fruits were selected at harvest. They were peeled, cut in pieces, and 5 g samples were prepared, which were frozen in liquid nitrogen and stored at -20 ºC until analyses. Fruit samples were analyzed for sugars, vitamin C, total phenolics, flavonoids, anthocyanins content, and antioxidant capacity as described by Abidi et al. (2011). For the determination of ascorbic acid the samples were kept frozen in 5% metaphosphoric acid and homogenized at 4 ºC. Then, they were centrifuged at 16,000 rpm for 20 min at 4 ºC, and the supernatant was immediately used for vitamin C analysis. Absorbance was measured at 525 nm and the amount of vitamin C was expressed as mg of ascorbic acid (AsA) per 100 g fresh weight (FW) (Zaharieva and Abadía 2003). For the determination of sugars, at the moment of analysis the frozen fruit material (5 g) was homogenised in a Polytron (2 min on ice) with 10 mL of extraction solution consisting of 800 mL L−1 ethanol/Milli-Q water. The mixture was centrifuged at 16,000 rpm for 20 min at 4 ºC. The supernatant was recovered and assayed by high-performance liquid chromatography (HPLC) as described by Cantín et al. (2009a). For volatiles compounds, the head space solid phase microextraction was the method used for capturing volatiles. For head space analysis, a 65 μm polydimethylsiloxane–divinylbenzene (PDMS/DVB) SPME fiber (Supelco Inc. Bellefonte, PA, USA) was used (Sánchez et al. 2012).
2.3. Statistical analyses
All statistical analyses were performed using SPSS 19.0 (SPSS Inc., Chicago, IL). Data for each genotype in the four years of study were averaged, and mean values were used as estimated genotypic values.
3. Results and Discussion
3.1. Antioxidant compounds
The results showed a high variability among genotypes indicating that genetics plays an important role on these fruit quality traits (Table 1). The ascorbic acid content ranged in the progeny from 1.1 to 12.1 mg of AA/100g of FW (Table 1). Values were in the same range as previously reported for vitamin C contents in peach flesh, namely, 1-14 mg of AA/100 g of FW (Tavarini et al. 2008). The amounts of total phenolics (Table 1) fell within the range reported in the literature for peach fruits, namely 14–77 mg GA/100 g of FW (Proteggente et al. 2002). Flavonoids presented a mean value of 12.6 mg CE/100g of FW (Table 1) and fell within the range reported by Cantín et al. (2009b) from 1.8 to 30.9 mg of CE/100 g of FW. The anthocyanins content showed a normal distribution and ranged from 0.3 to 25 mg C3G eq/kg of FW (Table 1). Lavelli et al. (2009) reported that the cyanidin 3-O-glucoside in peach samples ranged from 0 to 9.4 mg/kg. The relative antioxidant capacity (RAC) ranged from 125.3 to 1099.6 (μg Trolox/g of FW) (Table 1). Cantín et al. (2009b) reported that the RAC varied among genotypes, with values ranging from 227.3 to 629.9 μg of Trolox/g of FW, with an average of 405 μg of Trolox/g of FW.
Table 1. Antioxidant compounds in the “Venus” × “Big Top” population. |
|||||
Progenitors |
Progeny |
||||
Traits |
“Venus” |
“Big Top” |
Min |
Max |
Mean ± SE |
Vitamin C |
3.0 ± 0.7 |
4.9 ± 0.7 |
1.1 |
12.1 |
4.1 ± 0.1 |
Total phenolics |
22.1 ± 8.0 |
26.4 ± 9.8 |
12.1 |
58.8 |
32.2 ± 0.9 |
Flavonoids |
7.6 ± 3.8 |
7.8 ± 4.6 |
1.6 |
60.1 |
12.6 ± 0.6 |
Anthocyanins |
2.1 ± 0.1 |
5.9 ± 2.2 |
0.3 |
25.7 |
3.1 ± 0.2 |
RAC |
386.1 ± 18.5 |
521.4 ± 47.4 |
125.3 |
1099.6 |
451.4 ± 11.7 |
Units: Vitamin C (mg AsA/100 g of FW); Total phenolics (mg GAE/100 g of FW); Flavonoids (mg CE/100 g of FW); Anthocyanins (mg C3GE/kg of FW); RAC; Relative Antioxidant Capacity (μg TE/g of FW). Abbreviations: AsA = Ascorbic acid; C3GE = Cyanidin-3-glucoside equivalents; CE = Catechin equivalents; GAE = Gallic acid equivalents; TE = Trolox equivalents.
3.2. Sugar content
The studied population exhibited wide phenotypic variations in sugar contents among genotypes (Table 2). Total sugars (the sum of sucrose, glucose, fructose and sorbitol contents) ranged from 45 to 205 mg/g of FW. Cantín et al. (2009a) reported that the average content of total sugars in the peeled nectarines fruit was 77.1 mg/g FW. Sucrose was the major sugar present in the evaluated genotypes, followed by fructose, glucose and sorbitol. Sorbitol content varied greatly among genotypes, ranging from 1.1 to 8.7 g kg−1 FW. Wu et al. (2005) reported that sucrose in peaches is dominant at maturity, followed by the reducing sugars (glucose and fructose) and then sorbitol.
Table 2. Total sugar content (g per kg FW) in the “Venus” × “Big Top” population. |
|||||
Progenitors |
Progeny |
||||
Traits |
“Venus” |
“Big Top” |
Min |
Max |
Mean ± SE |
Sucrose |
41.0 ± 5.7 |
85.1 ± 17.0 |
40.7 |
102.3 |
58.4 ± 1.2 |
Glucose |
10.0 ± 0.4 |
8.9 ± 0.8 |
8.3 |
23.4 |
12.2 ± 0.3 |
Fructose |
13.4 ± 0.5 |
10.9 ± 1.7 |
8.9 |
19.1 |
12.4 ± 0.2 |
Sorbitol |
8.6 ± 3.8 |
6.5 ± 1.7 |
1.7 |
19.5 |
6.6 ± 0.5 |
% Sucrose |
28.0 ± 2.5 |
38.1 ± 2.0 |
26.1 |
40.8 |
32.6 ± 2.8 |
% Glucose |
6.9 ± 1.5 |
4.0 ± 1.2 |
4.9 |
8.7 |
6.8 ± 1.1 |
% Fructose |
9.1 ± 1.3 |
4.9 ± 1.0 |
5.0 |
9.2 |
6.9 ± 1.0 |
% Sorbitol |
5.9 ± 0.5 |
2.9 ± 0.3 |
1.1 |
8.7 |
3.5 ± 0.2 |
Total sugars |
73.0 ± 9.6 |
111.5 ± 14.1 |
45.3 |
205.1 |
89.1 ± 1.5 |
3.3. Volatiles compounds
A total of sixty volatile compounds were identified and relatively quantified in the nectarine population (Table 3).
Most of these compounds have been previously reported in peach fruit by other authors (Zhang et al. 2010) and are included among the 100 or so volatile compounds already identified in peach and nectarine fruits. The broad ranges (relative quantification) and the normal distribution found for some lactones in the population have significance in peach breeding since volatile compounds can be genetically controlled and aroma plays a central role in fruit quality (Figure 1).
Sánchez et al. (2012) identified and quantified a total of 110 volatile compounds including: alcohols, ketones, aldehydes, esters, lactones, carboxylic acids, phenolics and terpenoids in peach fruit samples from different genetic backgrounds, locations and maturity stages. Almost all the volatile compounds showed dramatic changes in the levels of accumulation among the different progeny genotypes. Most reports in the literature have focused on the evolution of peach and nectarine aromas during ripening (Wang et al., 2009, and references therein). During fruit maturation, lactones with long side chain (γ-decalactone, δ-decalactone), benzaldehyde and linalool levels increased, reaching the highest amounts in mature fruit (Toselli et al., 2010 and references therein).
Table 3. List of the 60 volatile compounds detected in fruits of the nectarine ‘Venus’ x ‘Big Top’ population. |
|
Family |
Compounds |
Acids |
Butanoic |
|
Pentanoic |
|
Propenoic |
|
Hexanoic |
|
Acetic |
|
Heptanoic |
|
Valeric |
|
Octanoic |
|
Nonanoic |
|
Decanoic |
Alcohols |
Ethanol |
|
1-Pentanol |
|
1-Hexanol |
|
5-Methyl-5-octen-1-ol |
|
1,6-Octadien-3-ol |
Aldehydes |
Pentanal |
|
Hexenal |
|
Lilac aldehyde D |
|
Benzaldehyde |
|
Octanal |
|
2,4-Heptadienal |
|
Benzeneacetaldehyde |
|
Nonanal |
|
2-Nonenal |
|
2-Dodecenal |
Esters |
4-Hexen-1-ol, acetate |
|
Geranyl tiglate |
|
5-Methyl-3-phenylcyclopent-2-en-1-one |
Ketones |
3-Nonen-2-one |
|
5-Hepten-2-one |
|
4-Decanone |
|
Bicyclo[2.2.1]heptan-2-one |
|
m-Ethylacetophenone |
|
2-Buten-1-one |
|
But-3-en-2-one |
|
Geranyl acetone |
|
8,8,9-Trimethyl-deca-3,5-diene-2,7-dione |
|
5-Methyl-3-phenylcyclopent-2-en-1-one |
|
Benzophenone |
|
Methanone |
Lactones |
γ -Hexalactone |
|
γ -Heptalactone (2(3H)-Furanone |
|
γ -Octalactone (2(3H)-Furanone |
|
4-Methyl-5-penta-1,3-dienyltetrahydrofuran-2-one |
|
γ -Jasmolactone |
|
2H-Pyran-2-one |
|
γ -Decalactone |
|
δ-Decalactone |
Terpenoids |
cis- α -Terpineol |
|
β –Myrcene |
|
1,2-Cyclohexanediol |
|
p-Cymene |
|
Limonene |
|
Terpinolene |
|
Linalool |
|
Thymol |
|
α-Terpinol |
|
4-Hydroxy- α -ionone |
|
β-Damascenone |
|
β-Ionone |
|
Figure .1. Segregation of γ-Octalactone, β-Ionone, α-Terpinol and δ-Decalactone, in the ‘Venus’ × ‘Big Top’ population. Data are means (n = 67-75 genotypes) of one year of study (2010). Arrows show the values for the parents ‘Venus’ (‘V’) and ‘Big Top’ (‘BT’). |
4. Conclusion
Results indicate that nectarine fruit is a good source of phenolic compounds, vitamin C, sugar and volatile compounds and highlight the fact that ascorbic acid content is an important part of the overall evaluation of peach fruit quality. The comprehension of the biological mechanisms involved in the preventive action of these compounds on many chronic diseases must be elucidated to demonstrate their wide range of functions, many of which unrelated to the ability to absorb free radicals.
5. References
Abidi W, Cantín C, Jiménez S, Giménez R, Moreno MA, Gogorcena Y (2015) Influence of antioxidant compounds, total sugars and genetic background on the chilling injury susceptibility of a non-melting peach (Prunus persica (L.) Batsch) progeny. J. Sci. Food Agric 95: 351-358
Abidi W, Jiménez S, Moreno MÁ, Gogorcena Y (2011) Evaluation of antioxidant compounds and total sugar content in a nectarine [Prunus persica (L.) Batsch] progeny. Inter. J. Mol. Sci 12 (10): 6919-6935
Block G, Patterson B, Subar A (1992) Fruit, vegetables, and cancer prevention: a review of the epidemiological evidence. Nutr. Cancer 18 (1): 1-29
Cantín C, Gogorcena Y, Moreno M.A (2009a) Analysis of phenotypic variation of sugar profile in different peach and nectarine [Prunus persica (L.) Batsch] breeding progenies. J. Sci. Food Agric 89: 1909-1917
Cantín C, Moreno M.A, Gogorcena Y (2009b) Evaluation of the antioxidant capacity, phenolic compounds, and vitamin C content of different peach and nectarine [Prunus persica (L.) Batsch] breeding progenies. J. Agric. Food Chem 57: 4586-4592
Chai Q, Wu B, Liu W, Wang L, Yang C, Wang Y, Fang J, Liu Y, Li S (2012) Volatiles of plums evaluated by HS-SPME with GC–MS at the germplasm level. Food Chem 130: 432-440
Dauchet L, Dallongeville J (2008) Fruit and vegetables and cardiovascular disease: epidemiological evidence from the non-Western world. Brit. J. Nutr 99(02): 219-20
Infante R, Farcuh M, Meneses C (2008) Monitoring the sensorial quality and aroma through an electronic nose in peaches during cold storage. J. Sci. Food Agric 88: 2073-2078
Islam MR, Afrin S, Ahmed Khan T, Hossain Z (2015) Nutrient content and antioxidant properties of some popular fruits in bangladesh. Inter.J.Pharm.Sci.Research 6(4): 1407-1414
Karadag A, Ozcelik B, Saner S (2009) Review of methods to determine antioxidant capacities. Food Anal. Methods 2: 41-60
Lavelli V, Pompei C, Casadei MA (2009) Quality of nectarine and peach nectars as affected by lye-peeling and storage. J. Agric. Food Chem 115: 1291-1298
Proteggente AR, Pannala AS, Paganga G (2002) The antioxidant activity of regularly consumed fruit and vegetables reflects their phenolics and vitamin C composition. Free Radical Res 36: 217-233
Sánchez G, Besada C, Badenes ML, Monforte A, Granell A (2012) A non-targeted approach unravels the volatile network in peach fruit. PLoS ONE 7(6): e38992
Tavarini S, Degl’Innocenti E, Remorini D, Massai R, Guidi L (2008) Preliminary characterisation of peach cultivars for their antioxidant capacity. J. Food Sci. Tech 43: 810-815
Tsantili Eleni, Shina Y, Nocka JF, Watkinsa CB (2010) Antioxidant concentrations during chilling injury development in peaches. Postharvest. Biol. Technol 57: 27-34
Wolfe KL, Kang X, He X, Dong M, Zhang Q, Liu RH (2008) Cellular antioxidante activity of common fruits. J. Agric. Food Chem 56: 8418-8426
Wu BH, Quilot B, Génard M, Kervella J, Li SH (2005) Changes in sugar and organic acid concentrations during fruit maturation in peaches, P. davidiana and hybrids as analyzed by principal component analysis. Sci. Hort. 103: 429-439
Zaharieva T, Abadía J (2003) Iron deficiency enhances the levels of ascorbate, glutathione and related enzymes in sugar beet roots. Protoplasma 221: 269-275
Zhang Q, Yan G, Dai H, Zhang X, Li C, Zhang Z (2008) Characterization of Tomentosa cherry (Prunus tomentosa Thunb.) genotypes using SSR markers and morphological traits. Sci. Hort 118: 39-47
Zhang B, Shen JY, Wei W, Xi WP, Xu CJ, Ferguson I, Chen K (2010) Expression of genes associated with aroma formation derived from the fatty acid pathway during peach fruit ripening. J. Agric. Food Chem 58 (10): 6157-6165