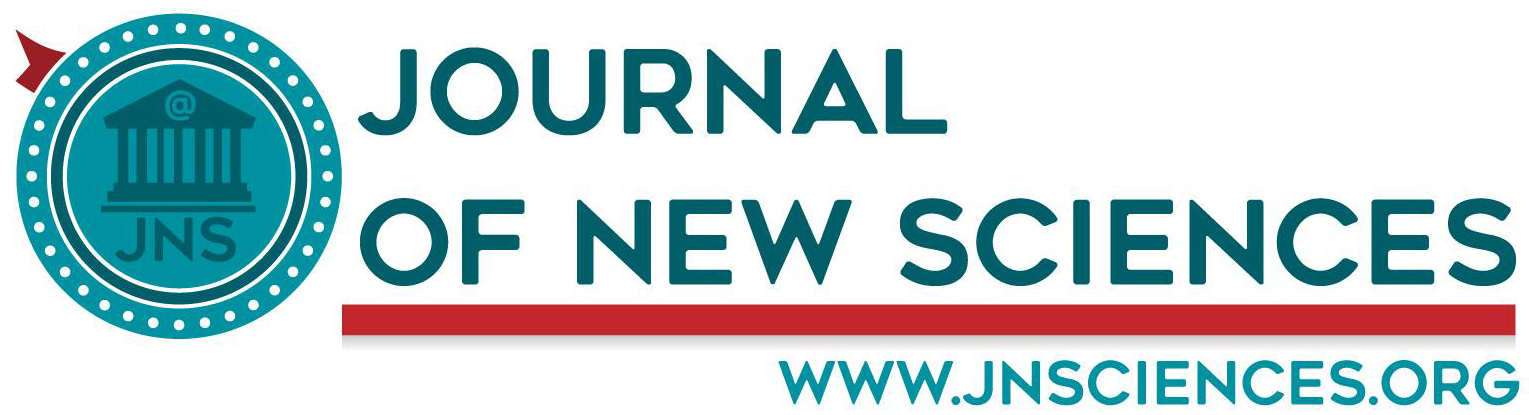
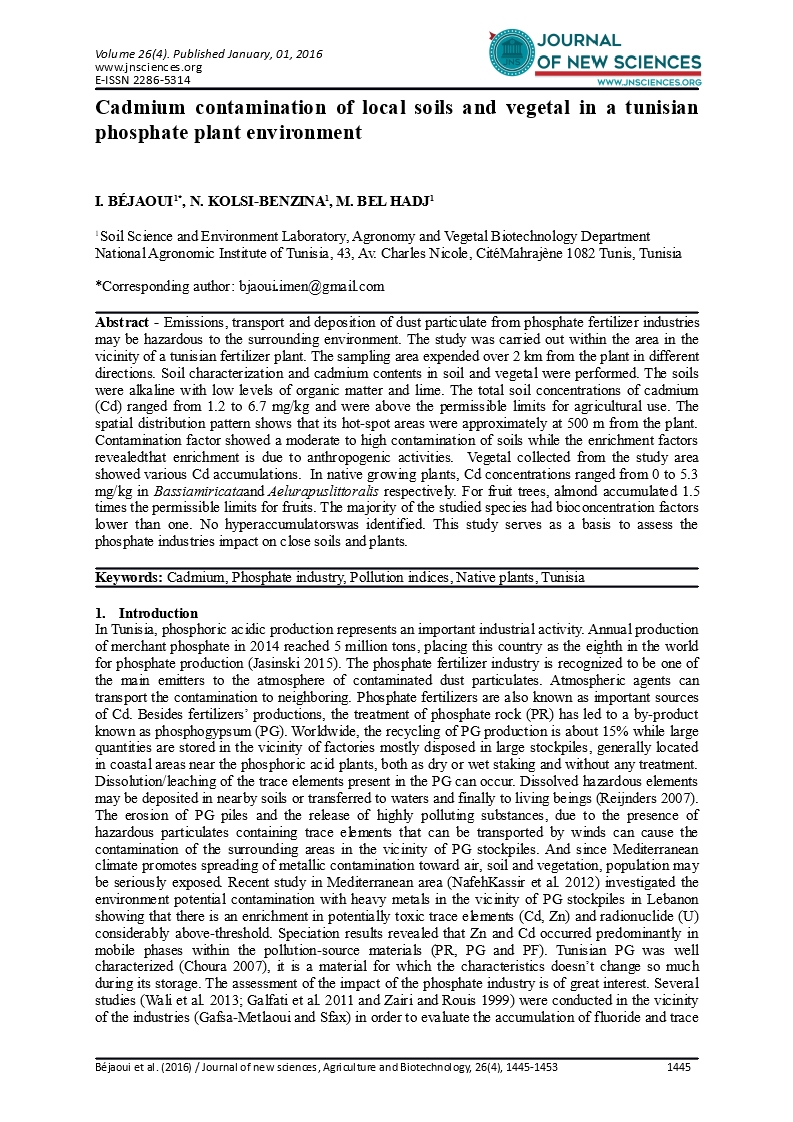
- Category: Volume 26
- Hits: 18257
Cadmium contamination of local soils and vegetal in a tunisian phosphate plant environment
I. Béjaoui1*
N. Kolsi-Benzina1
M. Bel Hadj1
1Soil Science and Environment Laboratory, Agronomy and Vegetal Biotechnology Department National Agronomic Institute of Tunisia, 43, Av. Charles Nicole, CitéMahrajène 1082 Tunis, Tunisia
Abstract - Emissions, transport and deposition of dust particulate from phosphate fertilizer industries may be hazardous to the surrounding environment. The study was carried out within the area in the vicinity of a tunisian fertilizer plant. The sampling area expended over 2 km from the plant in different directions. Soil characterization and cadmium contents in soil and vegetal were performed. The soils were alkaline with low levels of organic matter and lime. The total soil concentrations of cadmium (Cd) ranged from 1.2 to 6.7 mg/kg and were above the permissible limits for agricultural use. The spatial distribution pattern shows that its hot-spot areas were approximately at 500 m from the plant. Contamination factor showed a moderate to high contamination of soils while the enrichment factors revealedthat enrichment is due to anthropogenic activities. Vegetal collected from the study area showed various Cd accumulations. In native growing plants, Cd concentrations ranged from 0 to 5.3 mg/kg in Bassiamiricataand Aelurapuslittoralis respectively. For fruit trees, almond accumulated 1.5 times the permissible limits for fruits. The majority of the studied species had bioconcentration factors lower than one. No hyperaccumulatorswas identified. This study serves as a basis to assess the phosphate industries impact on close soils and plants.
Keywords: Cadmium, Phosphate industry, Pollution indices, Native plants, Tunisia
-
Introduction
In Tunisia, phosphoric acidic production represents an important industrial activity. Annual production of merchant phosphate in 2014 reached 5 million tons, placing this country as the eighth in the world for phosphate production (Jasinski 2015). The phosphate fertilizer industry is recognized to be one of the main emitters to the atmosphere of contaminated dust particulates. Atmospheric agents can transport the contamination to neighboring. Phosphate fertilizers are also known as important sources of Cd. Besides fertilizers’ productions, the treatment of phosphate rock (PR) has led to a by-product known as phosphogypsum (PG). Worldwide, the recycling of PG production is about 15% while large quantities are stored in the vicinity of factories mostly disposed in large stockpiles, generally located in coastal areas near the phosphoric acid plants, both as dry or wet staking and without any treatment. Dissolution/leaching of the trace elements present in the PG can occur. Dissolved hazardous elements may be deposited in nearby soils or transferred to waters and finally to living beings (Reijnders 2007). The erosion of PG piles and the release of highly polluting substances, due to the presence of hazardous particulates containing trace elements that can be transported by winds can cause the contamination of the surrounding areas in the vicinity of PG stockpiles. And since Mediterranean climate promotes spreading of metallic contamination toward air, soil and vegetation, population may be seriously exposed. Recent study in Mediterranean area (NafehKassir et al. 2012) investigated the environment potential contamination with heavy metals in the vicinity of PG stockpiles in Lebanon showing that there is an enrichment in potentially toxic trace elements (Cd, Zn) and radionuclide (U) considerably above-threshold. Speciation results revealed that Zn and Cd occurred predominantly in mobile phases within the pollution-source materials (PR, PG and PF). Tunisian PG was well characterized (Choura 2007), it is a material for which the characteristics doesn’t change so much during its storage. The assessment of the impact of the phosphate industry is of great interest. Several studies (Wali et al. 2013; Galfati et al. 2011 and Zairi and Rouis 1999) were conducted in the vicinity of the industries (Gafsa-Metlaoui and Sfax) in order to evaluate the accumulation of fluoride and trace elements in soils and plants. A study on samples of groundwater and soils from two sites in Sfax (NPK and SIAPE) up to150 meters from the PG piles showed the presence of fluorine pollution, phosphates and trace elements exceeding the levels of Tunisian standards on solid wastes (Zairi and Rouis 1999). Wali et al. (2013) showed the existence of a medium to highly polluted zone with trace elements (Zn, Pb, Cr, Cu, Co and Ni) surrounding the Tunisian phosphate industry (SIAPE) and that the source of soil contamination was anthropogenic industrial activities in the region of Sfax on the Mediterranean coast, 270 km south of Tunis City. The aim of this study is to evaluate accumulation of cadmium (Cd), considered as being one of the most ecotoxic metals that exhibit adverse effects on all biological processes of humans, animals, and plants. This metal has lower retention to mineral phases of soils and is contained in PG particulates emitted to the atmosphere on the surrounding soils then observed in native growing plants in the vicinity of this industry. Soil Cd content and plant Cd content are compared to the threshold values. Thus, the specific objectives include: 1) to study the horizontal distribution of cadmium; 2) to assess the extent of anthropogenic contribution in soil Cd contamination and 3) to assess the Cd bioconcentration factor in an attempt to understand the plants ability to accumulate cadmium.
-
Materials and Methods
-
Study area description
The study area is located in Sfax, southern Tunisia. Subject to a continental and maritime influence, the region is characterized by a semi-arid to arid climate. Recorded annual precipitations, over 60 year’s period, give an average rainfall of about 237.8 mm. The prevailing winds are south or south-easterly, wind speeds are generally medium to low, with an average of 9 m.s-1, up to 15 m.S-1 and a minimum of 5 m.S-1 (AGS 2013). The soils are generally sandy or sandy-limestone. The dominant crops are olive groves and orchards occupying 80% of agricultural lands, followed by cereals with 4.5%. The considered phosphate plant has been processing raw materials for an extended period of time sufficient to make the assessment of its impact on the surrounding environment reliable. Dust emissions by the plant are estimated annually to 1610 tons. Furthermore, according to Azri (2000), the TSP unit of the plant discharges into the atmosphere a very high heavy metal flows (e.g. 653 kg Cd/year) and the particles of cadmium oxide (Ø <1 µm) falls out up to 5 km from the fertilizer industry. The plant has two piles of PG. The first one started in the 1950s is currently operational and covers a 52 ha and the amount of stored PG is 15 million tons. The second one, served for storage from 1974 up to 1990 is 12 m height and has a total volume of 5.3 million m3 PG. Solid particles are carried by wind, as well as acids and washed particles (Choura 2007).
-
Soilsampling and preparation
The sampling design adopted in the studied area consists of four marked line transects. The soil samples (n=12), tagged from A to D indicating the directions and followed by the distance from the fertilizer industry (Figure 1). On each direction, the sites are 0.5 km, 1 km, and 2 km distant from the PG pile. We attributed the distance as an index number.
|
Figure 1. Sampling sites along 4 axes A, B, C and D |
The samples were collected in a systematically depth of 0-20 cm for all sites. Each soil sample was prepared by first randomly collecting several sub-samples distanced by 1 m around each sampling site, followed by thoroughly mixing of the sub-samples to form a composite sample of 1 kg using quartile method. A representative sample of the PG pile was also collected. The sample, collected in plastic bags, were brought to laboratory, air-dried and passed through a sieve of 2 mm and properly stored for analysis.
-
Vegetal sampling and preparation
The vegetal samples were collected from the same sites from where the soil samples were collected. Areal part samples of native growing plants (n=9) and locally grown fruit trees (n=5) were collected (Table 3). After washing with distilled water to remove soil particles and dried (70 °C, 24 h) they were crushed for analysis in three replicates.
-
Analytical procedures
The soil fraction of 2 mm was used to determine pH (1:2.5 soil water extract) using a pH meter and electric conductivity (CE) (1:5 soil water extract). The soil organic carbon content was found by the K2Cr2O7 digestion of Walkley- Black method. The value of soil organic matter (SOM) was obtained by multiplying organic carbon by 1.724 (Nelson and Sommers, 1982). For the total Cd content, soil samples were digested according to NF X 31-415 method (nitric acid and hydrochloric acid). Vegetal samples were powdered and 1 g was heated in the oven at 450°C for 2 hours. After cooling for 20 minutes, the samples were filtered using 0.2 microns filter, supplemented with ultrapure water before dosing atomic absorption. Each preparation of sample was repeated in triplicate (Pauwels et al., 1992).
-
Indices of pollution
Assessment of metal and its contamination level in soils require pre-anthropogenic knowledge of metal concentrations to act as pristine values. A number of different enrichment calculation methods and different reference material have been reported (Dragovanić et al. 2008;Harikumar and Jisha 2010). In this study, the enrichment factor (EF) and the contamination factor (CF) were applied to assess heavy metal contamination in soil located in the vicinity of the fertilizer industry.
-
Contamination Factor (CF)
The level of contamination of soil by metal is expressed in terms of a CF calculated as:
(1)
Where, Cmsample is the mean metal concentration in soils and Cmbackground is the mean natural background value of that metal. CF < 1 refers to low contamination; 1 ≤ CF < 3 means moderate contamination; 3 ≤ CF ≤ 6 indicates considerable contamination and CF > 6 indicates very high contamination. Background value was estimated from the mean concentration of Cd for unaffected soil in the area as reported by Mezghani et al. (1999).
-
Enrichment Factor (EF)
EF is a factor that averages the accumulation of metal in soil samples in order to evaluate the degree of trace metal contamination in soils (Ferreira da Silva et al. 2005). EF can be calculated by averaging the ratios of the soil element concentrations in mg.kg-1 (Cm sample) to the permissible level (PL).
(2)
The permissible level employed for interpreting soil heavy contamination/pollution varies from country to country based on chosen factors. For our study, we will consider two values: PL1 is the element concentration in the soil, from which crops produced are considered as unsafe for human health (Cd = 3 mg/kg) (Kloke, 1979). The corresponding EF is noticed (EF1). PL2 is the permissible value for Cd in Tunisian garden soils and greenhouses (Cd = 2 mg/kg) (ANPE, 2013) and is used for EF2.
-
Bioconcentration factor (BCF)
The BCF which is defined as the ratio of metal concentration in the plants to that in soil was calculated as:
(3)
Where Cplants and Cmediumrepresent the heavy metal concentration in extracts of plants and soils on dry weight basis, respectively (Audet and Charest, 2007).
-
Statistical analysis
Statistical analysis was performed using the raw data. The measurements were expressed in term of mean and standard deviation. Relationships between metals and other controlling factors were determined by bivariate correlation using the Pearson coefficient in a two-tailed test (r < 0.01 and 0.05). All analysis was performed using SPSS software (version 16.0).
-
Results and discussion
-
PG and soils characterization
-
Mean values of physical and chemical characteristics of soil samples are shown in Table 1. Soil samples show a variable admixture of sand, silt and clay. Mean clay contents ranged from 19.5 to 42.5 %. Out of 13 samples analyzed, 7 samples belong to loamy clay sand textural class. Thus, it is obvious that soils of this study area are coarse to moderately coarse in texture.
Table 1. Chemical composition (Mean ± SD, n = 13) of PG and soil samples of the study area |
|||||
Sampling sites |
pH |
EC (mS/cm) |
SOM (%) |
Lime (%) |
% Clay |
0 (PG) |
3.2±0.03 |
2.9±0.03 |
0.8±0.01 |
nd |
nd |
A-0.5 |
8.6±0.1 |
1.02±0.02 |
0.6±0.06 |
2.5±0.2 |
23.6 |
A-1 |
8.7±0.1 |
1.4±0.05 |
0.4±0.02 |
2.4±0.08 |
22.2 |
A-2 |
9.2±0.02 |
9.1±0.02 |
1.6±0.07 |
13.8±0.4 |
26 |
B-0.5 |
8.5±0.03 |
0.3±0.03 |
1.4±0 |
2.05±0.08 |
29 |
B-1 |
8.7±0.1 |
0.1±0.01 |
0.2±0.01 |
3.1±0.1 |
19,5 |
B-2 |
8.4±0.14 |
0.2±0.1 |
0.5±0.02 |
3.1±0.1 |
21 |
C-0.5 |
8.04±0.2 |
1.16±0.02 |
0.6±0.02 |
2.5±0.08 |
25 |
C-1 |
7.9±0.01 |
1.01±0.02 |
0.2±0.01 |
1.4±0.06 |
32 |
C-2 |
8.5±0.1 |
0.8±0.03 |
0.3±0.02 |
3.1±0 |
33 |
D-0.5 |
8.2±0.03 |
1.2±0.03 |
2.4±0.05 |
5.7±0.3 |
42,5 |
D-1 |
8.7±0.3 |
0.7±0.08 |
0.01±0.01 |
7.7±0 |
38,5 |
D-2 |
8.8±0.1 |
1.4±0.16 |
2.1±0.08 |
6.9±0.2 |
40 |
SD: standard deviation; EC: electrical conductivity; SOM: Soil organic matter; nd: not determined |
For the PG pile (point 0), pH is highly acidic (3.19). This goes along with results of pH of Tunisian PG found by (Zairi and Rouis 1999). This acidity is due to an amount of acid, accounting for about 0.5 to 1% of treated P2O5 remained in the gypsum. Soil samples pH across the entire study area ranged in an interval varying from 7.9 to 9.2 with a mean value of pH of 8.6 ± 0.3, which indicates that the soils were alkaline. Soils pH increased significantly with the distance from the plant. The electrical conductivity (EC) decreased significantly with the distance from the plant. It was considerably higher for the PG pile (2.9 mS.cm-1) compared to soil samples on all axes except for A-2. Throughout the axis, the average total lime percentage is low and ranged from 2.3 to 6.7 %. The average soil organic matter (SOM) contents are low (0.4 to 1.3%). This variability is due to the great diversity of land use. Furthermore, the interaction “axis × distance from the plant” was statistically significant.
-
Cadmium analysis and pollution indices
The Cd content of the PG pile was 7.6 mg/kg and is in the range of Cd concentration of Tunisian PG as reported by Ben Amor and Jomaa (2012). For the soil samples, the mean values of Cd ranged from 1.33 to 6.67 mg/kgfor the four axes. Cd content decreased when the distance increases except at points B-2. The study area is a residential area but with a house garden system. The soils Cd concentrations were compared to the guideline value of Canada for residential and agricultural land uses, 10 and 1.4 mg/kg respectively (CCME 2007). Since the garden soils are used as agricultural lands and inhabitants consume their vegetal production, we will compare the results with agricultural permissible limits for Cd. Regarding these results, it is obvious that the concentrations of Cd in the PG surrounding area are much higher than in the reference soil except for D axis samples. This axis is south of the pile and prevalent winds are south ones. This result confirms wind action in the transport of contaminant particles from the plant.
High Pearson correlations between soil properties and soil cadmium content are observed with pH and with the soil organic matter (SOM) (p<0.01 and p <0.05). This is indicative of the significance of soil pH and organic matter in the sorption of micro pollutants in soil. Although the importance of SOM in the Cd fixation is proven, cadmium mobility is significantly correlated mainly with pH. No correlation was found between the Cd content and EC or between Cd and percent lime.
The contamination factor (CF) and enrichment index (EI) are presented in Table 2.
Table 2. Mean soil Cd (ppm), contamination factor (CF) * and enrichment indexes (EF)* classes of Cd studied with respect to the natural background of 0.7 ppm Cd and 2 permissible limits (PL1= 3 ppm Cd for EF1 and PL2= 2 ppm Cd for EF2)
|
||||
Sampling sites |
Cd (ppm) |
CF |
EF1 |
EF2 |
0 |
7.6 |
10.9 |
2.5 |
3.8 |
A-0.5 |
6.7 |
9.5 |
2.2 |
3.3 |
A-1 |
5.6 |
8.07 |
1.9 |
2.8 |
A-2 |
4.7 |
6.7 |
1.5 |
2.3 |
B-0.5 |
3.6 |
5.14 |
1.2 |
1.8 |
B-1 |
2.6 |
3.7 |
0.9 |
1.3 |
B-2 |
4.4 |
6.2 |
1.46 |
2.2 |
C-0.5 |
4.5 |
6.5 |
1.5 |
2.3 |
C-1 |
4.4 |
6.27 |
1.46 |
2.3 |
C-2 |
3.4 |
4.9 |
1.1 |
1.16 |
D-0.5 |
1.3 |
1.9 |
0.44 |
0.66 |
D-1 |
1.2 |
1.7 |
0.4 |
0.6 |
D-2 |
nd |
nd |
nd |
nd |
*Values calculated based on soil layer 0 - 20 cm only, nd: not detected |
The assessment of the contamination of the studied agricultural soils was based on CFs (Eq. 1). The CFs were very high ranging from 1.9 to 10.9. This result indicates that the contamination varies from mean to very high. The high values of the CFs (>1) in all soil samples can be due to influence of industrial activities.
EF (Eq. 2) was used in the study to assess the relative contributions of natural and anthropogenic heavy metal inputs to soils. Details of the EF values of Cd with respect to the permissible limits concentrations are presented in Table 2. According to Chen et al. (2007), EF< 1 indicated no enrichment, EF <3 is minor enrichment, EF=3-5 is moderate enrichment. Besides, EF values between 0.5 and 1.5 indicate the metal is entirely from crustal materials or natural processes, whereas EF values greater than 1.5 suggest that the sources are more likely to be anthropogenic. The calculated EFs compared with the permissible limit of Cd in soils from which crops produced are considered as unsafe for human; there is a minor enrichment for all soil samples (EF < 3) from anthropogenic sources. Considering the (PL2) suggested in Tunisia, there is a moderate enrichment from anthropogenic sources for the pile sample and for the site A-0.5 (500 meters from the PG stock pile) and a minor enrichment for the other sampling sites. There is no enrichment for soil sampled on axis D since it’s on the opposite direction of the prevalent wind.
-
Cadmium uptake by plants
The uptake of trace elements in plant depends on plant species and on metal availability in the soil. The absorption of trace elements by plants can be passively with the mass flow of water through the roots (main entry route) or through leaf stomata after atmospheric depositions. Under normal growing conditions, plants can potentially accumulate some metal ions in order of magnitude greater than the surrounding medium (Yoon et al. 2006). In this study, a total of 16 vegetal species was collected from the sites. We distinguished two types of vegetal, spontaneous plants and fruit trees, as trace metals are known to be accumulated to different degrees in different plant tissues (Douay et al. 2013).
A summary of the results of plant analyses for Cd is provided in Table 3. Total Cd concentrations in plants greatly vary and range from 0 to 5.3 (mg/kg DM), with the maximum in the leaves of Bassiamiricata grown on the PG pile, while the lowest concentration is in Aelurapuslittoralis and in Palm. Similar variation (0 - 6.6 mg/kg) was also present in the soil Cd concentrations. However, linear regression analysis (not shown) indicated no significant positive relationship between vegetal and soil Cd concentration. Furthermore, there was no significant relationship between Cd concentration in any of the vegetal types and soil pH, probably because the basic pH would act to strongly limit plant uptake.
Table 3. Mean Cd (mg.kg-1 DM) and bioconcentration factor (BCF) of Cd for sampled vegetal |
|||
Plants species |
Cd |
BCF |
Distance (m) from fertilizer plant |
Opuntiaficusindia |
0.7 |
0.09 |
0 |
Bassiamuricata (L.) |
5.3 |
0.69 |
0 |
Bassiamuricata (L.) |
2.3 |
0.34 |
500 |
Bassiamuricata (L.) |
2.3 |
0.49 |
500 |
Arecaceae |
0 |
0.00 |
500 |
Prunus amygdalus |
3.3 |
0.90 |
500 |
Prunus amygdalus (Fruit) |
1.3 |
0.35 |
500 |
Acacia cyanophylla |
2.3 |
0.49 |
500 |
Morus |
1.0 |
0.75 |
500 |
Prunus amygdalus |
2.0 |
0.75 |
1000 |
Prunus amygdalus |
2.0 |
1.50 |
1000 |
Asphodèle sp. |
3.3 |
0.58 |
1000 |
Stipa sp. |
1.7 |
1.28 |
1000 |
Olea europaea L. |
1.7 |
0.36 |
1000 |
Cynodondactylon |
2.3 |
0.49 |
1000 |
Casavenira |
3.0 |
0.64 |
2000 |
Atriplexsp. |
0.7 |
0.16 |
2000 |
Peganumharmala |
2.3 |
0.49 |
2000 |
Aelurapuslittoralis |
0.0 |
0 |
2000 |
The results of Cd concentrations in plants will be discussed based on the plant species and their behaviors towards metal accumulation, fruit trees on one hand and native growing plants on the other hand.
For fruit trees, leaves Cd concentration ranged from 0 to 3.3 mg/kg DM. The lowest leaves Cd concentration was for Palm and the highest was for Almond. Over all, Cd content was relatively lower than the phytotoxic level of 5 mg.kg-1 reported by Kabata-Pendias (2011). The Cd content in olive tree leaves (1.7 mg.kg-1 DM) at 1000 meters from the fertilizer plant was slightly higher than a previous reported concentration (1.57 mg/kg DM) at the same distance in the study area (Mezghani et al., 1999). This Cd content in olive tree leaves is 17 fold higher than the range reported in background areas under Mediterranean conditions that is 0.02–0.10 mg/kgDM (Bargagli, 1998). As the leaves and twigs of olive tree may constitute complementary feed for cattle and for wild animals, especially during the dry summer, this Cd concentration in olive leaves in such area is of great concern. Almond leaves had shown that Cd concentrations decreased with the distance. Similarly, Mezghani et al. (1999) reported a negative correlation between distance from the fertilizer plant and concentration of Cd in tree fruits. This uptake could be related to the important contribution of root uptake since cadmium is absorbed passively via the roots and freely translocated to the leaves. Only palm tree leaves had not accumulated Cd although the soil had Cd levels exceeded the permissible limits (6.6 mg/kgDM).
For native growing plants, and except for Aelurapuslittoralis, leaves Cd concentrations ranged from 0.05 to 5.3 mg/kg DM. All the species accumulate Cd more than the normal Cd plants contents set by Standard Reference Plant (0.05 mg/kg) (Birth 2003). However, these levels did not exceed the phytotoxic concentrations of Cd (Kabata-Pendias 2011) except for Bassiamuricata (L.) sampled on the PG pile. This latter vegetal accumulated high amount of Cd as reported by Galfati et al. (2011) near the plants and laundries of Gafsa Phosphates Company (CPG), and in the Gafsa- Métlaoui Basin. Besides, Peganumharmala and Acacia cyanophylla accumulate abnormal levels of Cd in their leaves which indicate their capacity to tolerate high concentrations of Cd. In fact, both species are suggested by several authors in phytoremediation of polluted soils (Masvodza et al. 2013 and Shah et al. 2013).
Herbaceous species, Cynodondactylon, accumulate Cd in their leaves (2.3 mg/kg DM). This result is consistent with the findings of Boularbah et al. (2006) indicating that this plant has the ability to accumulate heavy metals such as Cd, As and Sb in excessive amounts without adverse effects on the general development of the plant.
According to EPA definition in 2000, hyper accumulating plant is the plant that can concentrate over than 100 mg.kg of Cd in their shoots and roots. In this study, none of the plant species is hyper accumulator.
-
Bioconcentration factor (BCF)
The use of the content value of metal per plant or organ gives good estimation of heavy metal extraction efficiency in a given plant species. A plant's ability to accumulate metals from soils can be estimated using the BCF (Gupta and Sinha 2009). Many factors can influence the BCF, including soil properties and metals chemical phases. According to Yoon et al., (2006), BCF can be used to estimate a plant's potential for phytoremediation purposes.
The BCF (Eq. 3) results shown in Table 3 indicate that of the fourteen studied plant species, Palm and Aelurapuslittoralisare non-accumulator plants (BCF< 0.01); Opuntiaficusindia is a low accumulator plant (0.01< BCF < 0.1); Bassiamuricata (L.), Almond , Acacia cyanophylla, Morus, Asphodèle sp., Olive Cynodondactylonand Peganumharmala are moderate accumulator plants (0.1< BCF < 1). The plants exhibiting a BCF < 1 are unsuitable for phytoextraction (Fitz and Wenzel 2002).
The majority of the studied plants exhibited a BCF greater than 0.5 which indicate that there is a great chance of Cd vegetal contamination by anthropogenic activities and a hazard to animal or human contamination. So the need for environmental monitoring of this area is highly required.
-
Conclusion
The present study investigated on the potential hazard of Tunisian phosphate fertilizer industry emissions on soil and plant contamination with Cd. Assessment of pollution in soils in the vicinity of the fertilizer phosphate treatment plant in Sfax was evaluated by total Cd contents and by using contamination factor and enrichment factors. Degree of enrichment was examined by comparing soil cadmium contents obtained using background concentration and permissible limits obtained from literature. The level of contamination is affected by close proximity of the sampling sites to the industry. Generally, the average content of Cd was higher than the international guidelines values for agricultural soils criteria. The contamination factor showed that generally there is moderate or high contamination of Cd across the sampling sites. Enrichment factor ratios showed that the soils were deficiently to minimally enrich by Cd. This is a reflection of anthropogenic contribution which might partly result from the fertilizer plant and point emission sources. The contents of Cd were also analyzed in plants sampled from the surrounding area of the fertilizer industry. The metal accumulation in plants in our sites was compared to the common levels for trace elements(Cd) and the results showed that the contents of this element in the studied plants vary between species, the values were high but no significant positive relationship between vegetal and soil Cd concentration is shown. The results indicated that Bassiamuricata (L.) was the specie with the most leaves Cd accumulation. Arecaceaeand Aelurapuslittoralisdid not accumulate Cd in their leaves. In this present work, the majority of the studied species had BCF lower than one. None of the plants were suitable for the phytoextraction because no hyperaccumulator was identified. Based on the results of this study mobility and bioavailability of Cd in soil and the potential of using studied plants for soil phytostabilisation may be possible but need further studies.
-
References
Adriano DC, (2001) Trace Elements in Terrestrial Environments: Biogeochemistry, Bioavailability, and Risks of Metals, 2nd edition, Springer Verlag, New York.
ANPE, (2013) Etude détaillée des risques. Manuel version 02 (07/05/2013). Annexe 2 : Evaluation chimique, Agence Nationale de Protection de l’Environnement, Tunis, 3-10.
AGS, (2013)Atlas du Gouvernorat de SfaxDirection de l’aménagement du territoire, (in French) On line at : http://www.mehat.gov.tn/index.php?id=347&L=1
Audet P. and Charest C., (2007) Heavy metal phytoremediation from a meta-analytical perspective. Environmental Pollution, 147, 231-237.
Azri C, Maalej A, Medhioub K, (2000) Etude de la variabilité des constituants de l'aérosol dans la ville de Sfax (Tunisie). Pollution Atomsphérique, 165, 121-129.
Bargagli R, (1998) Trace elements in terrestrial plants: an ecophysiological approach to biomonitoring and biorecovery. Berlin7 Springer- Verlag. 324.
Ben Amor F, Jomaa S, (2012) Reforme politique concernant la gestion du phosphogypse en Tunisie (Activité 2.1.1). Phase 1:evaluation de la situation actuelle, UNEP (DEC)/MED WG.202/2. 45.
Boularbah A, Schwartz C, Bitton G, Aboudrar NW, Ouhammou A, Morel JL, (2006) Heavy metal contamination from mining sites in South Morocco: 2. Assessment of metal accumulation and toxicity in plants. Chemosphere, 63, 811-817.
Canadian Council of Ministers of the Environment, (2007) Canadian soil quality guidelines for the protection of environmental and human health: Summary Tables. Update 7.0. On line at: http://www.ec.gc.ca/ceqg-rcqe
Chen CW, Kao CM, Chen CF, Lao C, (2007) Heavy metals and metalloids in sediments from the Llobregat basin, Spain, Environmental Geology, 44, 325-332.
Choura M, (2007) Short and medium action program III-Tunisia: Environmental evaluation of the treatment of phosphate in the south coastal zone of Sfax. Municipally of Sfax, Internal report, Tunisia.
Douay F, Pelfrêne A, Planque J, Fourrier H, Richard A, Roussel H, Girondelot B, (2013) Assessment of potential health risk for inhabitants living near a former lead smelter. Part 1: metal concentrations in soils, agricultural crops, and homegrown vegetables. Environmental Monitoring and Assessment, 185, 3665-3680.
Dragović S, Mihailović N, Gajić B, (2008) Heavy metals in soils: distribution, relationship with soil characteristics and radionuclides and multivariate assessment of contamination sources. Chemosphere, 74, 491-495.
Ferreira da Silva E, Matos JX, Patinha C, Reis P, Cardoso Fonseca E, (2005) The effect of unconfined mine tailings on the geochemistry of soils, sediments and surface waters of the Lousal area (Iberian Pyrite Belt, Southern Portugal). Land Degradation and Development, 16, 213–228
Fitz WJ, Wenzel WW, (2002) Arsenic Transformation in the Soil– Rhizosphere–Plant System, Fundamentals and Potential Application of Phytoremediation, Journal of Biotechnolog, 99 (3), 259-278.
Galfati I, Essaïd B, BejiSassi A, Abdallah H, Zaïer A, (2011) Accumulation of heavy metals in native plants growing near the phosphate treatment industry, Tunisia. Carpathian Journal of Earth and Environmental Sciences, 6 (2), 85-100.
Gupta AK, Sinha S, (2009) Growth and metal accumulation response of Vignaradiata L. var PDM 54 (Mung bean) grown on fly ash amended soil: Effect on dietary intake. Environmental Geochemistry and Health, 1 (4), 463-73.
Harikumar PS, Jisha TS, (2010) Distribution pattern of trace metal pollutants in the sediments of an urban wetland in the southwest coast of India. International Journal of Engineering Science and Technology, 2 (5): 540–850.
Jasinski SM, (2015) Phosphate rock. U.S. Geological Survey, Mineral Commodity Summaries, 703, 118-119.
Kabata-Pendias A, (2011) Trace Elements in Soil & Plants, CRC Press, Taylor and Francis Group LLC, 4th edition, Boca Raton, USA, 365p.
Kloke A, (1979) Contents of arsenic, cadmium, chromium, fluorine, lead, mercury, and nickel in plants grown on contaminated soil. UN-ECE Symposium, Geneva.
Masvodza DR, Dzomba P, Mhandu F, Masamha B, (2013) Heavy Metal Content in Acacia saligna and Acacia polyacantha on Slime Dams: Implications for Phytoremediation. American Journal of Experimental Agriculture, 3 (4), 871-883.
Mezghani I, Boukhris M, Chaieb M, (1999) Accumulation du cadmium par quelques espèces végétales cultivées aux environs d'une usine d'engrais phosphatés à Sfax (Tunisie), Pollution Atmosphérique, 80-88.
NafehKassir L., Lartiges B., Ouaini N., (2012) Effects of fertilizer industry emissions on local soil contamination: A case study of phosphate plant on the east Mediterranean coast. Environmental Technology, 33 (8), 873-885.
Nelson, PW and Sommers CE, (1982) Total Carbon, Organic Carbon and Organic Matter. In: Page, A.L., Ed., Methods of Soil Analysis, Part 2, SSSA, Madison, 539-579.
Pauwels JM., Van Ranst E, Verloo M, Mvondo ZA, (1992) Manuel de laboratoire de pédologie: Méthodes d’analyse de sol et des plantes. Equipement, gestion des stocks de verreries et produits chimiques. Publication agricole, 28, 250.
Reijnders, L, (2007) Cleaner phosphogypsum, coal combustion ashes and waste incineration ashes for application in building materials, A review. Building and Environment, 42 (2), 1036-1042.
Shah A, Niaz A, Ullah N, Rehman A, Akhlaq M, Zakir M, Khan MS, (2013) Comparative Study of Heavy Metals in Soil and Selected Medicinal Plants. Journal of Chemistry, Article ID 621265, 5 pages.
Sponza D, Karaoglu N, (2002) Environmental geochemistry and pollution studies of Aliaga metal industry district. Environment International, 27(7), 541-53.
Wali A, Colinet G, Khadhraoui M, Ksibi M, (2013) Trace Metals in Surface Soil Contaminated by Release of Phosphate Industry in the Surroundings of Sfax-Tunisia. Environmental Research, Engineering and Management 3 (65), 20-30.
Yoon Y, Cao X, Zhou Q , Ma LQ, (2006) Accumulation of Pb, Cu, and Zn in native plants growing on a contaminated Florida site. Science of Total Environment, 368, 456-464.
Zairi M et Rouis M, (1999) Impacts environnementaux du stockage du phosphogypse à Sfax (Tunisie), Bulletin des laboratoires des ponts et chaussées. REF 4145, 29-40.