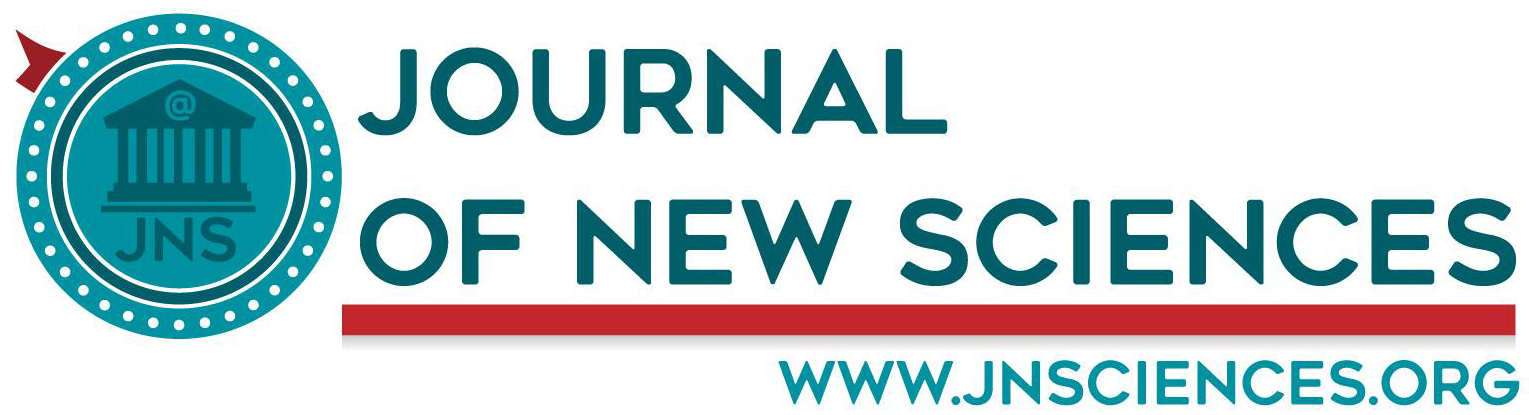
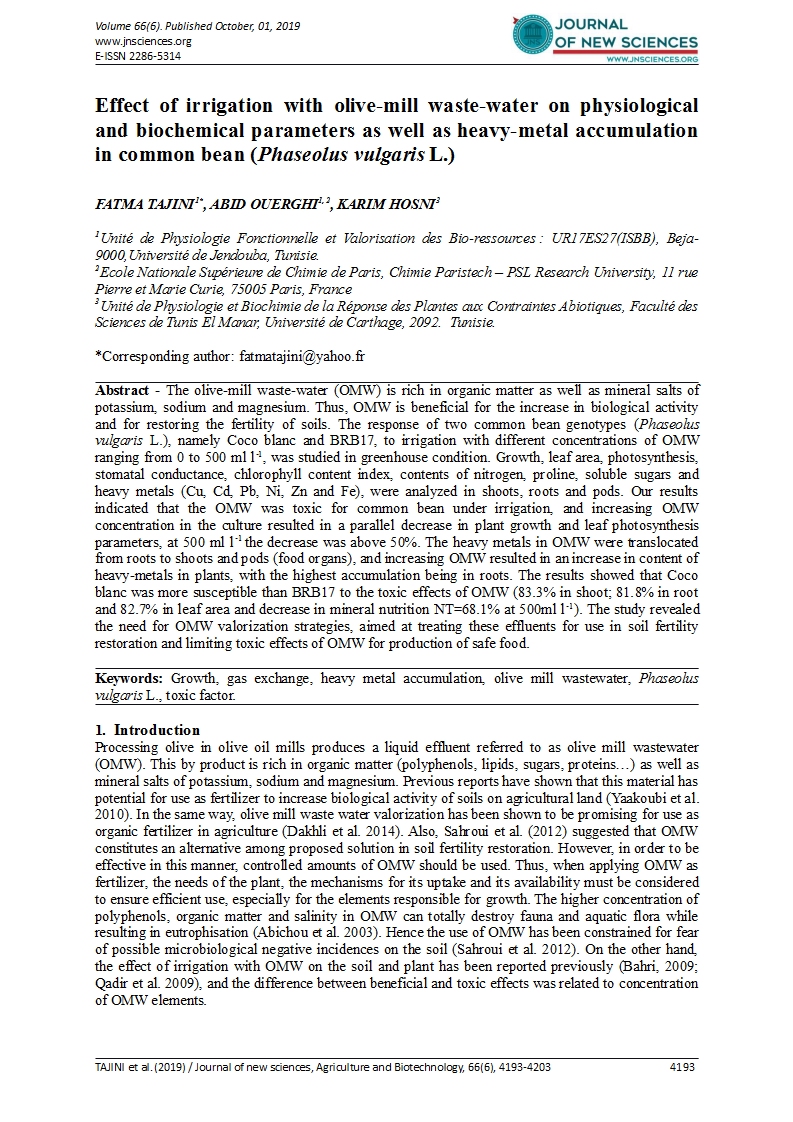
- Category: Volume 66
- Hits: 3297
Effect of irrigation with olive-mill waste-water on physiological and biochemical parameters as well as heavy-metal accumulation in common bean (Phaseolus vulgaris L.)
F. TAJINI1*
A. OUERGHI1, 2
K. HOSNI3
1Unité de Physiologie Fonctionnelle et Valorisation des Bio-ressources : UR17ES27(ISBB), Beja- 9000,Université de Jendouba, Tunisie.
2Ecole Nationale Supérieure de Chimie de Paris, Chimie Paristech – PSL Research University, 11 rue Pierre et Marie Curie, 75005 Paris, France
3Unité de Physiologie et Biochimie de la Réponse des Plantes aux Contraintes Abiotiques, Faculté des Sciences de Tunis El Manar, Université de Carthage, 2092. Tunisie.
Abstract - The olive-mill waste-water (OMW) is rich in organic matter as well as mineral salts of potassium, sodium and magnesium. Thus, OMW is beneficial for the increase in biological activity and for restoring the fertility of soils. The response of two common bean genotypes (Phaseolus vulgaris L.), namely Coco blanc and BRB17, to irrigation with different concentrations of OMW ranging from 0 to 500 ml l-1, was studied in greenhouse condition. Growth, leaf area, photosynthesis, stomatal conductance, chlorophyll content index, contents of nitrogen, proline, soluble sugars and heavy metals (Cu, Cd, Pb, Ni, Zn and Fe), were analyzed in shoots, roots and pods. Our results indicated that the OMW was toxic for common bean under irrigation, and increasing OMW concentration in the culture resulted in a parallel decrease in plant growth and leaf photosynthesis parameters, at 500 ml l-1 the decrease was above 50%. The heavy metals in OMW were translocated from roots to shoots and pods (food organs), and increasing OMW resulted in an increase in content of heavy-metals in plants, with the highest accumulation being in roots. The results showed that Coco blanc was more susceptible than BRB17 to the toxic effects of OMW (83.3% in shoot; 81.8% in root and 82.7% in leaf area and decrease in mineral nutrition NT=68.1% at 500ml l-1). The study revealed the need for OMW valorization strategies, aimed at treating these effluents for use in soil fertility restoration and limiting toxic effects of OMW for production of safe food.
Keywords: Growth, gas exchange, heavy metal accumulation, olive mill wastewater, Phaseolus vulgaris L., toxic factor.
1. Introduction
Processing olive in olive oil mills produces a liquid effluent referred to as olive mill wastewater (OMW). This by product is rich in organic matter (polyphenols, lipids, sugars, proteins…) as well as mineral salts of potassium, sodium and magnesium. Previous reports have shown that this material has potential for use as fertilizer to increase biological activity of soils on agricultural land (Yaakoubi et al. 2010). In the same way, olive mill waste water valorization has been shown to be promising for use as organic fertilizer in agriculture (Dakhli et al. 2014). Also, Sahroui et al. (2012) suggested that OMW constitutes an alternative among proposed solution in soil fertility restoration. However, in order to be effective in this manner, controlled amounts of OMW should be used. Thus, when applying OMW as fertilizer, the needs of the plant, the mechanisms for its uptake and its availability must be considered to ensure efficient use, especially for the elements responsible for growth. The higher concentration of polyphenols, organic matter and salinity in OMW can totally destroy fauna and aquatic flora while resulting in eutrophisation (Abichou et al. 2003). Hence the use of OMW has been constrained for fear of possible microbiological negative incidences on the soil (Sahroui et al. 2012). On the other hand, the effect of irrigation with OMW on the soil and plant has been reported previously (Bahri, 2009; Qadir et al. 2009), and the difference between beneficial and toxic effects was related to concentration of OMW elements.
Diaz and Massol-Deya (2003) reported that translocation of heavy metal depends mainly on the botanical species. So, the transfer of some metals in different organs of the plant is a very limited phenomenon and depends on concentration, such is the case with Pb. Its accumulation in roots is only beyond 1000 ppm in the soil. Some heavy metals like Cu induced a decrease in chlorophyll content by inhibition of iron absorption (Patsikka et al. 2002) ; yet others such as Cd and Cr induced inactivation of the enzyme involved in the biosynthesis of the chlorophyll pigments and decrease in plant growth (Nallely et al. 2016; Barreiro et al. 2004; Jianrong and Qiran 2009 ).
The aim of the present study was to assess, the impact of various OMW concentrations on morphological, physiological and biochemical parameters and heavy metal accumulation in common bean (Phaseolus vulgaris L.)
2. Materials and methods
2.1. Test material
Two common bean (Phaseolus vulgaris L.) cultivars were used in this study: Coco blanc was selected as a pure line from the local cultivar Coco; and BRB17 was chosen on the basis of its adaptability to Mediterranean conditions (Trabelsi and Sifi, 1997). These cultivars were selected from a collection initially supplied by B. Voyesset from CIAT (Colombia). Olive mill wastewater was collected from an oil-works (region of Elkef). It was filtered to remove the non-soluble suspension materials and different concentrations were prepared for irrigation. The pH was estimated to be 5.2. The heavy metal constituents of OMW are listed in Table 1.
Table 1: Heavy metals concentration in olive mill wastewater (OMW). |
|
Analysis metal |
Concentration (mg/l) |
Cu |
0.093 |
Cd |
0.003 |
Pb |
0.107 |
Ni |
0.059 |
Zn |
1.239 |
Fe |
25.937 |
2.2. Plant growth conditions
The experiment was carried out in a greenhouse in 10 L pots filled with soil-peaty, sand, and gravel in a ratio of 5:4:1(v:v:v). The main soil-peaty characteristics are listed in Table 2.
Seeds were surface-sterilized with 1.3% calcium hypochloride for 15 min with constant stirring and subsequently washed with sterile distilled water. They were germinated on 0.8% sterile agar plates for 3 days at 28°C in the dark, with a germination rate of 80%.
Trials were performed in a temperature-controlled greenhouse with night/day temperatures of 20 ± 2°C / 25 ± 5°C, and relative humidity of 75/55 ± 10% and natural illuminations. When the trifoliate leaf appeared approximately 21 days after sowing (DAS), plants were distributed into 5 treatments: the first one was irrigated with distilled water (control) and the others were watered once a week with 10, 50, 250 and 500 ml l-1 of OMW solutions (that is 2, 10, 50 and 100 ml/200ml/plant).
There were four replicates for each genotype and each treatment with one plant only per pot. Pots were irrigated with 100 ml distilled water every 2 days until harvest, and the pots were distributed in a completely randomized design (CRD).
Table 2: Chemicals and physical properties of the soil-peaty |
|
pH 1/2.5 |
7.1 ± 0.2 |
EC mhom/cm (1/5) |
1.6 ± 0.3 |
Organic matter (%) |
1.2 ± 0.3 |
K2O assim (ppm) |
103 ± 7 |
Olsen P2O5 (ppm) |
182 |
Total nitrogen (ppm) |
595 |
Cl- |
8.1 ± 0.5 |
Na+ |
7.7 ± 0.6 |
K+ |
1.0 ± 0.3 |
Mg++ |
3.1 ± 0.4 |
Ca++ |
4.2 ± 0.3 |
SO42- |
7.1±0.3 |
2.3. Photosynthesis parameters
Measurements were taken at pod formation stage and after nine weeks of growth. Leaf chlorophyll content index was measured using Chlorophyll Content Meter, (Opti-Sciences, CCM 200). The readings were taken between 10:00 and 11:30. The mean of 10 readings per plant was used. Net photosynthesis and stomatal conductance were measured using (LCiPro) (ADC BioScientific Ltd).The readings were recorded forenoon between 10:00 and 11:30. Gas exchange measurements were performed on fully expanded and sun-exposed leaves throughout the natural photoperiod. A mean of 10 readings per plant was used.
2.4. Leaf area and biomass determination
The plants were harvested after nine weeks of growth. The estimation of leaf area was carried out using a planimeter to directly measure the leaf area (cm2) of detached leaves treated as screens to light rays. The shoots and roots were separated and dried at 80°C for 2 days, and the dry weight of each fraction was determined.
2.5. Determination of nitrogen, proline, soluble sugars and heavy metal
Total nitrogen (TN) in shoots was measured by the Kjeldahl procedure. The accumulation of proline was determined according to the method described by Khedr et al. (2003). Total soluble sugars were estimated by the phenol sulphuric acid method (DuBois et al. 1965).
Heavy metal contents were measured in treatment plants according to the method of Zorrig et al. (2010) with some modifications. Briefly, for each treatment, plant samples were dried at 80 °C for 48 h; and 1 g of each crushed fraction of shoots, roots and pods were incubated in 1N H2SO4 at 37°C for 12 hours, then 30 ml of distilled water was added to each solution. After filtration, concentrations of Cd, Cu, Pb, Ni, Zn and Fe in the extracts were determined by Inductively Coupled Plasma (ICP, Horiba Jobin-Yvon, Ultima 2CE.).
Before application of OMW treatments, we estimated no heavy metals in soil-peaty, except with very low concentration for Fe (0.0007 ppm) and Zn (0.0001 ppm), according to the method of Andersson (1976).
2.6 Statistical analysis
The experimental design consisted of a completely randomized design with 4 replications. The SAS software was used to perform the statistical analyses, and results were subjected to one way ANOVA, and comparison of means was achieved by the Duncan's multiple range test (p ≤ 0.05).
3. Results
3.1. Effect of OMW on leaf photosynthesis and stomatal conductance
In order to analyze the effects of OMW on plant growth, genotypes were exposed over 45 days to 0, 10, 50, 250 and 500 ml l-1 of OMW. The two genotypes exhibited similar overall response with respect to OMW toxicity symptoms. Exposure to increasing OMW concentrations resulted in a reduction of both leaf photosynthesis and stomatal conductance (Figure 1). All the plants subjected to 500 ml l-1 OMW exhibited symptoms of chlorosis, whereas at 250 ml l-1 OMW, chlorosis was far less.
Above the concentration of 10 ml l-1, the decrease in photosynthesis and stomatal conductance was above 50% for both genotypes. Indeed, irrigation with OMW caused a significant decrease in photosynthesis of 55.5; 75.3 and 79.4% for Coco blanc and 58.2; 78.4 and 82.4% for BRB17 at concentrations 50; 250 and 500 ml l-1, respectively (Figures 1A and 1B). Similarly, stomatal conductance was reduced to 54.1; 81.4 and 87.4% for Coco blanc and 65.9; 82.2 and 86.4% for BRB17 with the same concentrations (Figures 1C and 1D).
|
Figure 1 Effect of OMW on net photosynthesis (A, B) and stomatal conductance (C, D) of common bean genotypes Coco blanc and BRB17 grown in sand-soil culture and irrigated with 0, 10, 50, 250 and 500 ml l-1 concentrations of OMW. Data are means ± SD of 4 replicates (a mean of 10 readings per plant). Different letters indicate significant differences between treatment means according to Duncan's multiple range test (p ≤ 0.05). |
3.2. Effect of OMW on biomass yield
The two genotypes showed different levels of vigor under control conditions (no OMW added to the medium) (Figure 2). The two genotypes also had a comparatively different level of tolerance to OMW, with BRB17 having the most OMW tolerance to 50 ml l-1 (Figure 2).
OMW had an effect on the shoots, roots and leaf area. Interestingly, this effect was different depending on the concentrations. For BRB17, the presence of OMW induced no significant change in the biomass of shoots, roots and leaf area at 10 and 50 ml l-1, compared to the control plants. In contrast, in the same genotype, the shoots, roots and leaf area dropped from 65.5 to 85.5%; 70 to 72.1% and 68.3 to76.3%, respectively, when OMW concentration increased from 250 to 500 ml l-1 in the medium (Figures 2B, 2D and 2F).
In Coco blanc, irrigation with OMW induced a decrease of 32.6; 48.7 and 83.3% in shoots, 36.2; 75.0 and 81.8% in roots, as well as 30.2; 69.2 and 82.7% decrease in leaf area, under 50; 250 and 500 mll-1, respectively (Figures 2A, 2C and 2E).
|
Figure 2 Effect of OMW on shoots (A, B), roots (C, D) and leaf area (E, F) of common bean genotypes Coco blanc and BRB17 grown in sand-soil culture and irrigated with 0, 10, 50, 250 and 500 ml l-1 concentrations of OMW. Data are means ± SD of 4 replicates. Different letters indicate significant differences between treatment means according to Duncan's multiple range test (p ≤ 0.05). |
3.3. Effect of OMW on leaf chlorophyll content index and total nitrogen
We investigated whether OMW could impair mineral nutrition. Attention was focused on the analysis of total nitrogen. Total nitrogen in shoots decreased slightly in both common bean genotypes in response to increased OMW concentrations (Figures 3A and 3B). All OMW concentrations caused a less than 50% decrease in nitrogen content in both genotypes, except for 68.1% at 500ml l-1 for Coco blanc. However, chlorophyll content index was stable or increased for Coco blanc at 10 ml l-1. But, above this concentration, chlorophyll further decreased in both the genotypes (Figures 3C and 3D).
All OMW concentrations resulted in less than 50% decrease in chlorophyll content index for both genotypes, except for 58.2% at 500 ml l-1 for BRB17.
The decrease in the total nitrogen is likely a consequence of the decrease in the clorophyll accumulation and is probably a sign that nitrogen nutrition is markedly affected by the presence of OMW in the culture medium.
|
Figure 3 Effect of OMW on total nitrogen (A, B) and chlorophyll content index (C, D) of common bean genotypes Coco blanc and BRB17 grown in sand-soil culture and irrigated with 0, 10, 50, 250 and 500 ml l-1 concentrations of OMW. Data are means ± SD of 4 replicates. Different letters indicate significant differences between treatment means according to Duncan's multiple range test (p ≤ 0.05). |
3.4. Effect of OMW on leaf proline and soluble sugar contents
In contrast to the total nitrogen content, the proline content increased in shoots of both common bean genotypes in response to increases in OMW concentration (Figures 4A and 4B). The same result was observed with soluble sugar contents (Figures 4C and 4D).
In the control, the two common bean genotypes displayed comparable proline content. Accordingly, it was observed that Coco blanc, which displayed the highest proline contents, also had the highest soluble sugar contents in shoots, above 50ml l-1 (Figure 4).
|
Figure 4 Effect of Olive mill wastewater (OMW) on proline accumulation (A, B) and soluble sugars contents (C, D) of common bean genotypes Coco blanc and BRB17 grown in sand-soil culture and irrigated with 0, 10, 50, 250 and 500 ml l-1 concentrations of OMW. Data are means ± SD of 4 replicates. Different letters indicate significant differences between treatment means according to Duncan's multiple range test (p ≤ 0.05). |
3.5. Effect of OMW on heavy metal accumulation in shoots, roots and pods
Heavy metal content was measured in roots, shoots and pods of the two common bean genotypes. Unsurprisingly, the heavy metal contents of roots, shoots and pods increased almost linearly in response to increasing concentrations of OMW in the growth medium (Figure 5). However, the two genotypes displayed a differential ability to accumulate heavy metal in their roots as well as their shoots and pods. Except for nickel (Ni), the roots displayed the highest heavy metal content under all conditions. Pods displayed a different pattern; they displayed mainly low cadmium (Cd) content in Coco blanc, while showing generally no cadmium content in BRB17 genotype, in nearly all conditions. However, we observed the reverse with lead (Pb) content.
We examined whether the accumulation of these heavy metal in roots, shoots and pods could be related to different strategies with respect to OMW concentration. The two common bean genotypes displayed generally high heavy metal contents in roots, shoots and pods at 500 ml l-1 and mainly low heavy metal contents at 10 ml l-1 of OMW.
As expected, at 500 ml l-1, accumulation of heavy metal differed significantly (p≤0.001) between roots, shoots and pods. Root copper (Cu) content was at least twice as high as the content in shoots and pods (Figures 5A and 5B). By contrast, only traces of cadmium (Cd) were present in shoots and pods, compared to the Cd content in the roots of common bean genotypes (Figures 5C and 5D). In addition, lead (Pb) content was more than 4 times higher in roots than in shoots (Figures 5E and 5F), and the roots Ni content was at least twice as high as the shoots (Figures 5G and 5H). Zinc (Zn) content was more than 3 times higher in roots than in shoots and pods for Coco blanc, but it was more than twice as high in roots than in shoots for BRB17 (Figures 5I and 5J). Iron (Fe) content, however, was more than 10 and 6 times higher in roots than in shoots and pods for coco blanc and BRB17, respectively (Figures 5K and 5L).
4. Discussion
In the present study, we aimed to identify marked trends related to the response of common bean genotypes to the effects of OMW. To identify such trends, we comparatively analyzed two common bean genotypes, Coco blanc the local genotype in comparison to the tested genotype, namely BRB17, under irrigation with OMW in increasing concentrations. From this study, the first main trend found was that the OMW was a toxic factor for irrigation of common bean. Thus, the decrease in the stomatal conductance and photosynthesis in plants irrigated with increasing OMW concentrations (Figure1) could be related to the decrease in CO2 for the Calvin cycle (Flexas and Medrano 2002; Hessini et al. 2009). Photosynthesis is an original physiological process for the general metabolism of the cell. Any photosynthesis disturbance at the molecular level is connected with the low electron transport through PSII and/or with structural injuries of PSII and light harvesting complexes (Dubey 1997). Indeed, Munns et al. (2006) reported that a similar decrease of leaf area, in the presence of OMW, induced a decrease in translocation of CO2 in leaf area and consequently an inhibition of CO2 incorporation of this element and thus a negative effect on respiration. In addition, the decrease in photosynthesis (which is closely related to osmotic adaptation) was enhanced by the excessive accumulation of OMW element contents in plant cells (Hessini et al. 2009). If osmolyte accumulation in plant cells increases, osmotic potential decreases. This is due to stomatal closure, which restricts CO2 diffusion to chloroplasts (Muller and Whitsitt 1996), consequently as stomatal conductance decreases, photosynthesis is inhibited (Hessini et al. 2009).
The decrease in chlorophyll accumulation in plants irrigated by OMW could explain the decrease in photosynthetic activity; as it was found that there was a high correlation between both parameters (Ranjbar et al. 2002). Chlorophyll accumulation also had a positive correlation with nitrogen content; this was expected because nitrogen is one of the components of the chlorophyll molecule. The decrease of nitrogen content in the leaf reduces chlorophyll content, which in turn reduces the photosynthetic rate (Borrell and Hammer 2000; Bashir et al. 2007). On the other hand, a decrease in the chlorophyll content (Figure 3) under the influence of increasing concentrations of OMW could be explained by inhibition of chlorophyll synthesis by heavy metal contents (Figure 5) in these effluents (Hopkins 2003; Jianrong and Qiran 2009). In this context, heavy metal enhanced accumulation of phenol compound, which prevented plant cells from absorbing water and thus impaired mineral nutrition leading to chlorosis and a decrease in plant growth (Chaoui and El Ferjani 2004; Smeet et al. 2005).
|
Figure 5 Effect of OMW on heavy metal accumulation: Cu (A, B); Cd (C, D); Pb (E, F); Ni (G, H); Zn (I, J) and Fe (K, L) of common bean genotypes Coco blanc and BRB17 grown in sand-soil culture and irrigated with 10 (open bars), 50 (grey bars), 250 (hatched bars) and 500 ml l-1 (black bars) concentrations of OMW. Data are means ± SD of 3 replicates. For each organ, different letters indicate significant differences between treatment means according to Duncan's multiple range test (p ≤ 0.05). |
Our results indicate that accumulation of heavy metal in plant organs causes a greater decrease in plant growth of both common bean genotypes. This decrease was found at 50 and 250 ml l-1 OMW in Coco blanc and BRB17, respectively. Indeed, these results showed that Coco blanc was more susceptible than BRB17 to OMW.
The physiological, growth and developmental mechanisms which allow a plant species to tolerate toxic effects can involve numerous attributes. Indeed, our results suggest that a major factor controlling OMW tolerance in common bean is the ability to produce protective solutes, such as proline (Husen 2010) and soluble sugars (Elsheery and Cao 2008) to reduce the toxic effects of tissue heavy metal content. It is reported that total soluble sugars are the major solutes contributing to osmotic potential in plants (Zelalem et al. 2015), and they have different functions in plants from energy storage to signaling. Plants utilize several sugar-based strategies to adapt to environmental stresses (Anderson and Kohorn 2001; Chaves et al. 2003). Also, proline has been suggested to play multiple roles in plant stress tolerance. It acts as a mediator of osmotic adjustment, protects macromolecules during dehydration and serves as a hydroxyl radical scavenger (Parida et al. 2004). Thus, the genotype that showed the highest proline and soluble sugar content (Coco blanc) exhibited particularly typical OMW toxicity symptoms such as chlorosis and a decrease in plant growth (83.3% in shoot; 81.8% in root and 82.7% in leaf area and decrease in mineral nutrition NT=68.1% at 500ml l-1 ).
This sensitivity of plants to OMW was associated with excessive accumulation of heavy metal in leaves. This result is in agreement with the findings of Dakhli et al. (2014), who noticed that the application of OMW at high rates (100 m³/ha and 200 m³/ha) caused a significant reduction in growth of Hordeum vulgare, but doses of OMW less than 50 m³/ha did not have a significant effect on growth. Also, Yaakoubi et al. (2010) reported that application of OMW at 400 m3/ha decreased grain yield by 50 % in grass plants. On the contrary, other authors (Marsilio et al. 1990) indicated that there were no risks or particular problems, and that there is no danger of pollution of the groundwater with the application of OMW on agricultural lands (even in relatively high doses).
As OMW is rich in organic matter (polyphenols, lipids, sugars, proteins…) as well as in mineral salts of potassium, sodium and magnesium, it is likely to increase biological activity of soils and consequently their fertility following its spreading on agricultural land (Yaakoubi et al. 2010; Dakhli et al. 2014; Sahroui et al. 2012). However, OMW is also rich in heavy metal, using OMW as a soil fertilizer increased heavy metal in the soil culture. The second main trend emerging from this study is that the heavy metal in OMW were translocated from roots to shoots and pods in both genotypes; increasing OMW concentration in the medium resulted in a parallel increase in contents of heavy metals in roots, shoots and pods. Importantly, for each genotype, the highest content was displayed in the roots, which is likely a consequence of the accumulation of these metal in sand-soil culture (Friesl et al. 2004) and which probably induced their contamination by the effluent discharge, which in turn induced contamination of plant organs, especially pods (food organs) ,
5. Conclusion
Despite such a small sample, the magnitude of cultivated bean genotypes displayed a good basis to better understand mechanisms involved in the plant response to OMW. To some extent, our study revealed the significance for OMW valorization strategies, aimed at treating these effluents with physico - chemical or biologic treatments for their use in the restoration of soil fertility and improving agricultural production and limiting heavy metal accumulation in soil and their translocation in plant, inducing the toxic effects of OMW for safe food.
Acknowledgements
This manuscript is dedicated to all authors who contributed in this research.
6. References
Anderson C M, Kohorn B D (2001) Inactivation of Arabidopsis SIP1 leads to reduced levels of sugars and drought tolerance. Journal of Plant Physiology 158: 1215-1219.
Andersson A (1976) On the determination of ecologically significant fractions of some heavy metals in soils. Swedish Journal of Agricultural Research 6: 19-26.
Bahri A (2009) Managing the other side of the water cycle: making wastewater an asset. GWP Technical Committee 32: 1652-5369.
Barreiro O G, Rioboo C, Cid A, Herrero C (2004) Atrazine-Induced Chloorosis in Synechococcus elongates Cells. Archives of Environmental Contamination and Toxicology 46: 301-307.
Bashir F, Mahmooduzzafar T, Siddiqi O, Iqbal M (2007) The antioxidative response system in Glycine max (L.) Merr. exposed to Deltamethrin, a synthetic pyrethroid insecticide. Environmental Pollution147: 94-100.
Chaoui A, Elferjan E (2004) Impact of cadmium and copper excess on cell wall peroxidases in Pea stems. Pakistan Journal of Biological Sciences 7: 902-904.
Chaves M M, Maroco J P Pereira JS (2003) Understanding plant responses to drought-from genes to the whole plant. Functional Plant Biology 30: 239-264.
Dakhli R, Lamouri R, Maalej E M (2014) Effect of Olive mill waste water under salt stress conditions on phenological behavior of barley crop (Hordeum vulgare): Pot experiment). Journal of Materials and Environmental Science 5: 1033-1038.
Diaz E, Massol- Deya A (2003) Trace element composition in Forage samples from a military target range, three agricultural areas, and one natural area in Puerto Rico. Caribbean Journal of Science 39: 215-220.
Dubey R S (1997) Photosynthesis in Plants Under Stressful Conditions. In: Mohammad, P. (ed.), Handbook of Photosynthesis,University of Arizona, Tuscon, Arizona.
DuBois M, Gilles K, Hamilton J K, Rebers P A, Smith F (1965) Calorimitric method for determination of sugars and related substances. Analytical Chemistry 28:350-356.
Elsheery N I, Cao K F (2008) Gas exchange, chlorophyll fluorescence and osmotic adjustment in two mango cultivars under drought stress. Acta Physiologiae Plantarum 30: 769-777.
Flexa J, Mebrano H (2002) Drought–inhibition of photosynthesis in C3 plants: Stomatal and non-stomatal limitations revisited. Annals of Botany 89: 183-189.
Friesl W, Horak O, Wenzel W W (2004) Immobilization of heavy metals in soils by the application of bauxite residues: pot experiments under field conditions. Journal of Plant Nutrition and Soil Science 167: 54–59.
Hessini K, Martinez J P, Gandour M, Albouchi A, Soltani A Abdelly C (2009) Effect of water stress on growth, osmotic adjustment, cell wall elasticity and water-use efficiency in Spartina alterniflora. Environmental and Experimental Botany 67: 312-319.
Hopkins W G (2003) Physiologie végétale. Editions de Boeck Unversité, pp 514.
Husen A (2010) Growth characteristics, physiological and metabolic responses of teak (Tectona grandis L) clones differing in rejuvenation capacity subjected to drought stress. Silvae Genetica 59: 124-136.
Jianrong X, Qiran T (2009) Early stage toxicity of excess copper to photosystem II of Chlorella pyrenoidosa-0JIP chlorophyll a fluorescence analysis. Journal of Environmental Sciences 21: 1569-1574.
Khedr A A, Abbas M A, Abdel Wahid A A, Quickand W P, Abogadallah G M (2003) Proline induces the expression of salt-stress-responsive proteins and may improve the adaptation of Pancratium maritimum L. to salt-stress. Journal of Experimental Botany 54: 2553-2562.
Marsilio V, Giovacchino L D, Lombardo N, Briccoli-Bati C (1990) First observations on the disposal effects of olive mills vegetation waters on cultivated soil. Acta Horticulturae 286: 493-498.
Muller J E, Whitsitt M S (1996) Plant cellular responses to water deficit. Plant Growth Regulation 20: 41-46.
Munns R, Richard A J, Andre L (2006) Approches to increasing the salt tolerance of wheat and other cereals. Journal of Experimental Botany 57: 1025-1043.
Nallely T, Iván M, Alejandrodel P, Ingrid W, Juan H (2016) Cadmium phytoextraction capacity of white lupine (Lupinus albus L.) and narrow-leafed lupine (Lupinus angustifolius L.) in three contrasting agroclimatic conditions of Chile.Chilean journal of agricultural research76:228- 235.
Parida A K, Das A B, Sanad Y, Mohanty P (2004) Effects of salinity on biochemical components of the mangrove, Aegiceras corniculatum. Aquatic Botany 80: 77-87.
Patsikka E, Kairavuo M, Sersen F, Aro E M, Tystjarvi E (2002) Excess copper predispose photosystem II to photoinhibition in vitro by out competing iron and causing decrease in leaf chlorophyll. Plant Physiology 129: 1359-1367.
Qadir M, Bahri A, Toshio S, Al-Karadsheh E (2009) Waste water production, treatment, and irrigation in Middle East and North Africa. Irrigation and Drainage Systems 24: 27-51.
Ranjbar A, Van Damme P, Samson R, Lemeur R (2002) Leaf water status and photosynthetic gas exchange of P. khinjuk and P. mutica exposed to osmotic drought stress. Acta Horticulturae 591 : 423-428.
Sahroui H, Jrad A, Mellouli H J (2012) Épandage des margines sur les sols agricoles : impacts environnementaux microbiologiques. South African Journal of Science 8 : 97 – 106.
Smeet K, Cuypers A, Lambrecht A, Semane B, Hoet P, Laere A V, angronsveld J V (2005) Induction of oxidative stress and antioxidative mechanisms in Phaseolus vulgaris L. after Cd application. Plant Physiology and Biochemistry 43: 437-444.
Trabelsi M, Sifi B (1997) Screening for more performant common bean varieties. In:. Drevon, J.J. (ed.), Fysame Annual Report, INRA Montpellier, pp 23–33.
Yaakoubi A, Chahlaoui A, Rahmani M, Elyachioui M, Oulhote Y (2010) Effect of application of olive mill wastewater on soil microflora. Agrosolutions 20: 35-43.
Zelalem G, Azamal H, Masresha F, Gietahun Y (2015) Growth, Water Status, Physiological, Biochemical and Yield Response of Stay Green Sorghum (Sorghum bicolor L.) Varieties-A Field Trial Under Drought-Prone Area in Amhara Regional State, Ethiopian Journal of Agricultural Sciences 14: 188-202.
Zorrig W, Rouached A, Zaigham S, Abdelly C, Davidian J C, Berthomieu P (2010) Identification of three relationships linking cadmium accumulation to cadmium tolerance and zinc and citrate accumulation in lettuce. Plant Physiology 167: 1239–1247.