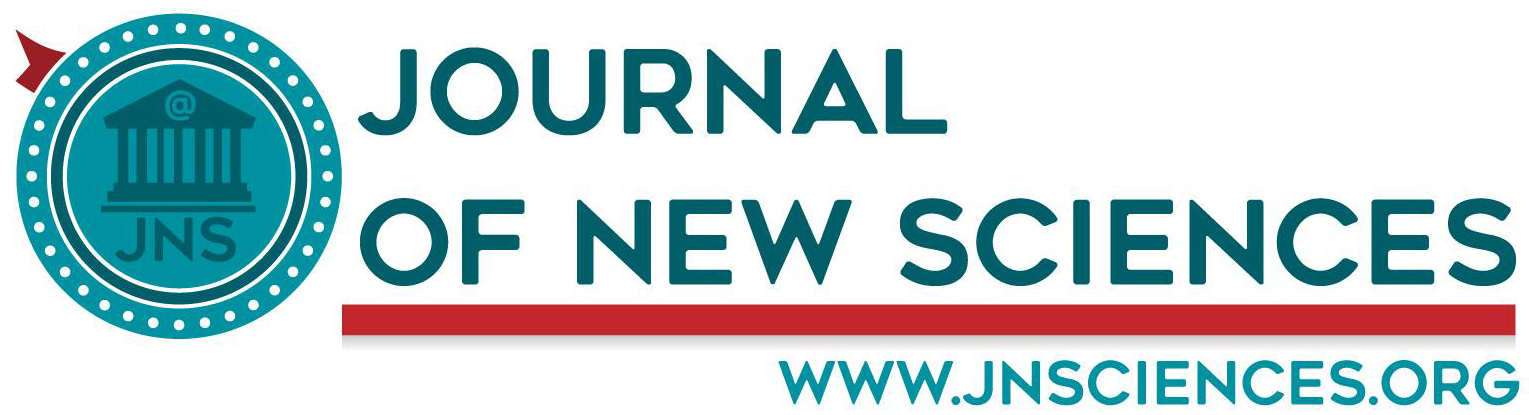
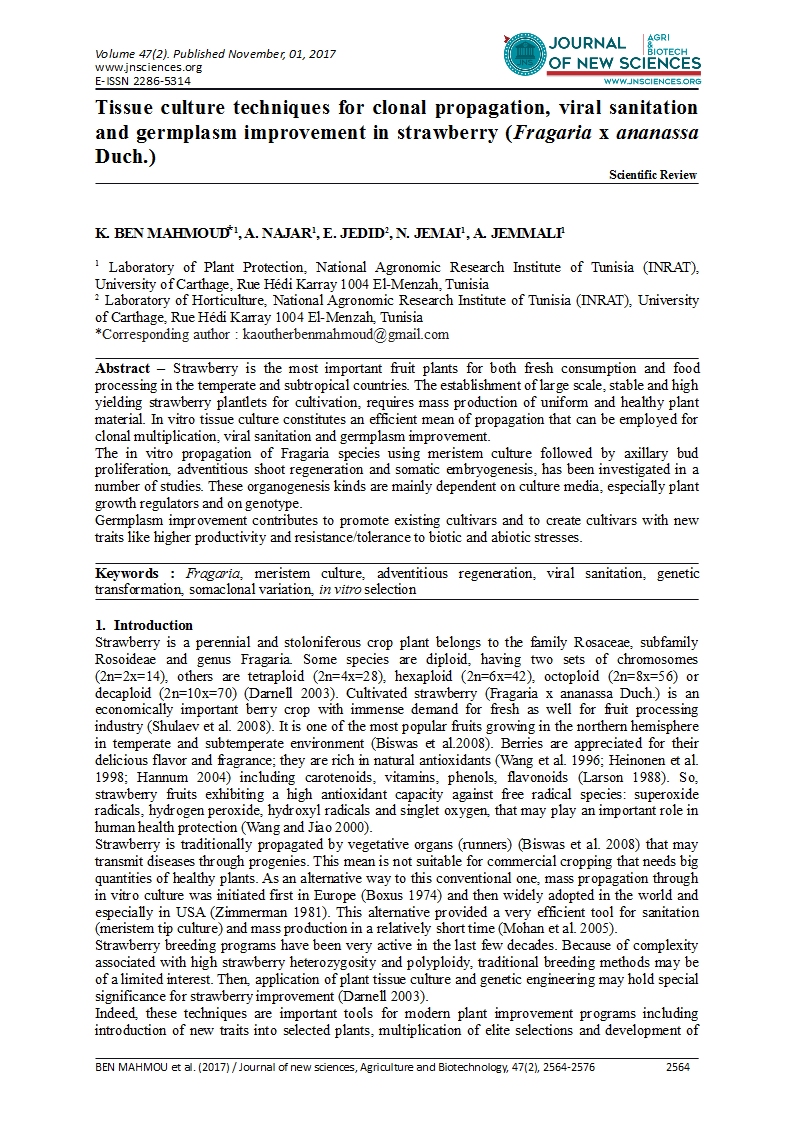
- Category: Volume 47
- Hits: 9453
Tissue culture techniques for clonal propagation, viral sanitation and germplasm improvement in strawberry (Fragaria x ananassa Duch.)
Scientific Review
K. BEN MAHMOUD⃰ 1
A. NAJAR1
E. JEDID2
N. JEMAI1
A. JEMMALI1
1 Laboratory of Plant Protection, National Agronomic Research Institute of Tunisia (INRAT), University of Carthage, Rue Hédi Karray 1004 El-Menzah, Tunisia
2 Laboratory of Horticulture, National Agronomic Research Institute of Tunisia (INRAT), University of Carthage, Rue Hédi Karray 1004 El-Menzah, Tunisia
Abstract – Strawberry is the most important fruit plants for both fresh consumption and food processing in the temperate and subtropical countries. The establishment of large scale, stable and high yielding strawberry plantlets for cultivation, requires mass production of uniform and healthy plant material. In vitro tissue culture constitutes an efficient mean of propagation that can be employed for clonal multiplication, viral sanitation and germplasm improvement. The in vitro propagation of Fragaria species using meristem culture followed by axillary bud proliferation, adventitious shoot regeneration and somatic embryogenesis, has been investigated in a number of studies. These organogenesis kinds are mainly dependent on culture media, especially plant growth regulators and on genotype. Germplasm improvement contributes to promote existing cultivars and to create cultivars with new traits like higher productivity and resistance/tolerance to biotic and abiotic stresses.
Keywords : Fragaria, meristem culture, adventitious regeneration, viral sanitation, genetic transformation, somaclonal variation, in vitro selection
1. Introduction
Strawberry is a perennial and stoloniferous crop plant belongs to the family Rosaceae, subfamily Rosoideae and genus Fragaria. Some species are diploid, having two sets of chromosomes (2n=2x=14), others are tetraploid (2n=4x=28), hexaploid (2n=6x=42), octoploid (2n=8x=56) or decaploid (2n=10x=70) (Darnell 2003). Cultivated strawberry (Fragaria x ananassa Duch.) is an economically important berry crop with immense demand for fresh as well for fruit processing industry (Shulaev et al. 2008). It is one of the most popular fruits growing in the northern hemisphere in temperate and subtemperate environment (Biswas et al.2008). Berries are appreciated for their delicious flavor and fragrance; they are rich in natural antioxidants (Wang et al. 1996; Heinonen et al. 1998; Hannum 2004) including carotenoids, vitamins, phenols, flavonoids (Larson 1988). So, strawberry fruits exhibiting a high antioxidant capacity against free radical species: superoxide radicals, hydrogen peroxide, hydroxyl radicals and singlet oxygen, that may play an important role in human health protection (Wang and Jiao 2000).
Strawberry is traditionally propagated by vegetative organs (runners) (Biswas et al. 2008) that may transmit diseases through progenies. This mean is not suitable for commercial cropping that needs big quantities of healthy plants. As an alternative way to this conventional one, mass propagation through in vitro culture was initiated first in Europe (Boxus 1974) and then widely adopted in the world and especially in USA (Zimmerman 1981). This alternative provided a very efficient tool for sanitation (meristem tip culture) and mass production in a relatively short time (Mohan et al. 2005).
Strawberry breeding programs have been very active in the last few decades. Because of complexity associated with high strawberry heterozygosity and polyploidy, traditional breeding methods may be of a limited interest. Then, application of plant tissue culture and genetic engineering may hold special significance for strawberry improvement (Darnell 2003).
Indeed, these techniques are important tools for modern plant improvement programs including introduction of new traits into selected plants, multiplication of elite selections and development of suitable cultivars, in short time. However, it was accepted that complementarity between classical breeding methods and new technologies would be better in quick and efficient achievement in genetic improvement (Cao and Hammershlag 2000).
The present review aims to give some highlights on (i) in vitro propagation via meristem culture for mass production and viral sanitation, (ii) regeneration via organogenesis and somatic embryogenesis and (iii) genetic improvement through somaclonal variation, in vitro selection and genetic transformation in strawberry (Fragaria x ananassa Duch.).
2. Micropropagation
Plant micropropagation is an “integrated process in which cells, tissues or organs of selected plants are isolated, surface sterilized and incubated in a growth-promoting aseptic environment to produce many clone plantlets”. The technique of cloning isolated single cells in vitro, demonstrated the fact that somatic cells, under appropriate conditions, may be able to differentiate into a whole plant. This potential of cells or tissues to form all cell types and regenerate a whole plant is the basic principle of plant cell totipotency (Steward et al. 1958).
Concerning strawberry, vegetative propagation is usually carried out via conventional method using runners to obtain true-to-type plant. When, this technique was used alone, it presented several limitations due to slowness, limited number of runners/plant/year and disease transmission through progenies. Coming of tissue culture techniques for strawberry propagation provided an alternative and novel possibility of producing a large number of virus-free plants from a single meristem-tip in a relatively short time and space (Fatemeh 2010).
The establishment of reliable and efficient protocols for plant micropropagation through meristem culture or through adventitious organogenesis (adventitious shoots or somatic embryos) from various explants could also make a significant contribution in improving strawberry culture (Fatemeh 2010).
2.1. Meristem culture
In vitro propagation through meristem culture is a secure clonal propagation method for true-to-type plant regeneration that guarantee stable transfer of desired traits into commercial genotypes without sexual recombination. It is also a powerful tool for large-scale propagation of plants (Kumar and Reddy 2011) or as a source of plant material for regeneration and transformation experiments (Boxus 1992). The explant used for strawberry micropropagation has been the meristem excised from runner tip (Sowik et al. 2001). A meristem with no leaf primordia or at most with 1-2 leaf primordia is excised and cultured in appropriate medium (Boxus 1974; Kumar and Reddy 2011). The ability of an apical shoot meristem to regenerate whole plant is the cornerstone of the micropropagation process (Debnath and Da Silva 2007).
Shoot tips are picked from young runners and meristems are removed and cultured ona nutrient medium with low level of cytokinins. A meristem consists in a dome of actively dividing cells, about 0.1 mm in diameter and 0.25 mm in long (Sowik et al. 2001). Regenerated explant is then cultured on a medium containing higher level of cytokinins to promote axillary budding without callus formation. Cytokinins are used to overcome apical dominance and enhance the branching of lateral buds from the leaf axis. Additional shoots are produced through further cycles of axillary bud branching (Debnath 2003). Cultures can be initiated and maintained on Boxus (Boxus 1974) medium containing Knop’s macronutrients and Murashige and Skoog (1962) micronutrients and organic components, or MS medium supplemented with BAP, IBA and GA3 (Cerović and Ružić 1989; Mozafari and Gerdakaneh 2012) (Table 1).
Several studies have attested that micropropagated plants show more advantages than those produced by conventional method in terms of fruit yield, vigor, number of runners per plant (Zebrowska et al. 2003) and number of leaves per plant (Karhu and Hakala 2002; Zebrowska et al. 2003). However, despite its extraordinary potential, this technology is still faced to some problems. For example, occurrence of morphological and yield variation in regenerated plants has been reported (Graham 2005). Variations consisted in dwarfism, chlorosis or white striping of leaves, multiapexing and stem fasciations, intensified vigour and abnormal flowering (hyper-flowering) accompanied by increased production of smaller fruits (Boxus 1999; Graham 2005).
These variants that caused problem for production of uniform, true-to-type plants, did not derive from meristems. Reported variations derived either from callus induced at the base of shoots (Nehra et al. 1994) or from stipular buds (without callus) (Jemmali et al. 1992, 1994). These stipular buds occurred spontaneously in vitro on strawberry regenerated from meristem culture and axillary proliferation. These stipular buds were cloned and their implication in hyper-flowering abnormality has been proved on cv. Gorella (Jemmali et al. 1992, 1994). Boxus (1999) underlined the risks caused by adventitious regeneration to the mass propagation systems of strawberry. To ovoid this, using lower BA concentration, shortening subculture duration and limiting number of subcultures were recommended (Jemmali et al. 1992; Graham 2005). Moreover, it has been proved that the application of GA3 delay the development of adventitious shoots (Boxus 1999; Litwińczuk and Zubel 2005). Gibberellic acid improved axillary shoot elongation and reduced callus growth as well as roots formation and adventitious shoots development (Litwińczuk et al. 2009).
Such practices may increase the costs of micropropagtion without full guarantee of plant conformity because adventitious proliferation is not easily detected by the in vitro procedures (Litwińczuk et al. 2009).
2.2. Adventitious organogenesis
Plant regeneration via adventitious shoots is a crucial aspect of plant biotechnology methods and tissue culture that facilitate the production of genetically engineered plants and somaclonal variants, and the rapid multiplication of difficult-to-propagate species (Debnath and Da Silva2007). This process is therefore a complex phenomenon, influenced by a number of genetic and environmental factors. Each species has its own specific requirements for in vitro regeneration. Fortunately, strawberry can be easily managed under in vitro conditions and efficient protocols for micropropagation and in vitro regeneration have been developed for many cultivars. This process is influenced by many factors such as ratio between plant growth regulators, culture conditions, genotype and explant type (Sorvari et al. 1993; Zhao et al. 2004; Hussaini and Abdin 2007).
Adventitious regeneration through direct or indirect regeneration has been obtained in many strawberry cultivars, using a board range of explants such as leaves (Jones at al. 1988; Nehra et al. 1989; Sorvari et al. 1993; Passey et al. 2003, Debnath 2005, 2006; Biswas et al. 2007; Karim et al. 2011), petioles (Foucault and Letouze 1987; Passey et al. 2003; Debnath 2005, 2006), flower buds (Foucault and Letouze 1987; Lis 1993), stipules (Rugini and Orlando 1992; Passey et al. 2003), roots (Rugini and Orlando 1992; Passey et al. 2003), runners (Liu and Sanford 1988; Lis 1993) (Table 1).
Growth and in vitro morphogenesis are regulated by the balance between the growth regulators supplied in the medium and the growth regulators produced endogenously (George et al. 2008). A balance in favor to cytokinin is often required for the formation of adventitious shoots.
In strawberry, most of regeneration protocols use Murashige and Skoog (1962) medium supplemented with 6-benzylaminopurine (BA) combined with auxins such as indole-3-butyric acid (IBA) (Quesada et al. 2007) or α-Naphthaleneacetic acid (NAA) (Karim et al. 2011). In recent years, there has been an increased interest on the use of thidiazuron (TDZ) instead of BA for strawberry regeneration. TDZ has been shown to be very effective on shoot regeneration of many species (Huetteman and Preece 1993), and its effect is not only due to its cytokinin like activity but also to its role as a modulator ofendogenous auxin levels (Quesada et al. 2007). Landi and Mezzetti (2006) evaluated the effect of TDZ on shoot regeneration from leaf tissue of several strawberry cultivars. A MS medium supplemented with TDZ and IBA in a temporary immersion bioreactor system has been used by Hanhineva et al. (2005) to optimize a protocol for shoot regeneration of five strawberry cultivars.
Genotype is also one of the most important factors affecting regeneration (Passey et al. 2003). The variation in the regeneration capacity among different cultivars has also been reported in some works (Jones et al. 1988; Nehra et al. 1989, 1990; Passey et al. 2003), indicating a strong genetic component determining the success of adventitious regeneration. It could be due, in part, to differences in levels of endogenous hormones, particularly cytokinin during the induction period; although the precise mechanism remains unclear (Schween and Schwenkel, 2003).
Type of explant is also one of the important factors in optimizing the tissue culture protocol. It significantly affects tissue culture process of plants (Kumar and Reddy 2011). In general, leaf tissue has the greatest regeneration capacity of all strawberry plant tissues in F. x ananassa (Passey et al. 2003; Jones et al. 1988; Popescu et al. 1997) and in wild strawberry F. vesca (El Mansouri et al. 1996; Alsheikh et al. 2002). Shoot regeneration rates using this explant are therefore very genotype-dependent.
Apart from growth regulators balance, genotype and type of explant, other factors can influence strawberry regeneration. Thus, Landi and Mezetti (2006) reported a stimulatory effect of continuous darkness incubation of explants on shoot regeneration. Dark incubation reduces tissue browning by inhibiting the enzymatic activity responsible for tissue oxidation (Titov et al. 2006). Besides this, it seems that a dark treatment for several weeks (Barceló et al. 1998; Hussaini and Abdin 2007) or even continuous darkness incubation (Landi and Mezetti, 2006) enhances organogenesis in strawberry leaf explants.
The addition of silver nitrate to the regeneration medium accelerates the initiation of adventitious buds and enhances shoot regeneration rates (Qin et al. 2005). This chemical has a significant stimulatory effect on chlorophyll and soluble protein contents as well as antioxidant enzyme activities. Tian et al. (2003) have found a correlation between endogenous antioxidant and morphogenetic process in strawberry.
2.3. Somatic embryogenesis
Somatic embryogenesis is the process by which somatic cells, under induction conditions, generate embryogenic cells, which go through a series of morphological and biochemical changes that result in the formation of somatic embryos (Quiroz-Figuera et al. 2006). The induction of somatic embryogenesis for in vitro plant regeneration provides more advantages than organogenesis (Wang et al. 2004). Somatic embryogenesis plays an important role in mass propagation, elimination of viruses, production of synthetic seeds (Swapnil et al. 2017) and conservation of germplasm via cryopreservation (Litz and Gray 1995; Merkle et al. 1995). When integrated with conventional breeding programs and molecular and cell biological techniques, it provides a valuable tool to enhance the genetic improvement of commercial crop species (Stasolla et al. 2003). Because somatic embryo resembles the zygotic embryo in various aspects (Feher et al. 2003), it may be used to study diverse subjects related to the embryogenesis process. Due to the presence of well-developed root and shoot primordia, somatic embryos germinated easily to produce plantlets without an additional step of rooting (Laux and Jugens 1997). Somatic embryos are defined as bipolar structures, which do not have a vascular connection with the mother tissue at any time of their life (Haccius 1978) and develop following the classical stages of embryogenesis (Williams and Maheswaran 1986).
Although plant regeneration through direct and indirect organogenesis has been reported in strawberry, only a few studies have so far focused on somatic embryogenesis (Donnoli et al. 2001; Biswas et al. 2007; Hussaini and Abdin 2007; Hussaini et al. 2008; Gerdakaneh and Zohari, 2013; Swapnil et al. 2017).
Several factors such as plant growth regulators combination, culture conditions influence embryogenic capacity of strawberry tissues. Plant growth regulators play a key role in the induction of somatic embryogenesis (Jimenez and Bangerth 2001). Particularly, auxins and cytokinins have been used to induce this phenomenon in strawberry. The following auxins 2,4-D (Stasolla et al. 2003; Kordestani and Karimi 2008), 4-amino-3,5,5-trichloro-2-pyridinecarboxylic acid (picloram) (Kordestani and Karimi 2008), indol-3-butyric acid (IBA) (Donnoli et al. 2001; Kordestani and Karimi, 2008) and α-naphtalene acetic acid (NAA) (Stasolla et al. 2003) used alone or in combination with the cytokinins BAP (Laux and Jugens 1997; Stasolla et al 2003) or thidiazuron (TDZ) (Pallavi et al. 2011) have been reported to induce embryogenesis (Table 1). Raemakers et al. (1995) estimated that in dicotyledonous species 2,4-D and BAP were the most used PGR for somatic embryo induction. Morphologically normal plants were obtained from somatic embryos that were transfered to MS medium containing NAA, GA3 and BA. Maintenance of the embryogenic cultures was, however, unsuccessful. Donnoli et al. (2001) reported somatic embryogenesis in 8% of the induced embryogenic calli in strawberry on MS medium supplemented with BA and IBA.
Light is an important culture condition that affects somatic embryogenesis during induction phase. Photoperiod has been implicated in the regulation of cytokinin levels as well as photoconversion of phytochromes (Torné et al. 1996).In strawberry, a negative effect of light on embryogenesis induction has been reported (Donnoli et al. 2001; Biswas et al. 2007). In chandler cultivar, a dark treatment significantly increased the number of somatic embryos on the leaf explants. Moreover, cold stress increased the embryogenic potential of strawberry (Hussaini and Abadin 2007).
Results of somatic embryogenesis in strawberry are still unsatisfying and more efforts are required to improve this technology (Swapnil et al. 2017).
Table 1. Main published results and corresponding references on strawberrymicropropagtionperformed techniques |
||||
Tissue culture technique |
cultivar |
Explants source |
Culture medium |
references |
micropropagtion via axillary shoots |
Kurdistan, Merk |
meristem |
N6 medium + BAP + IBA + GA3 |
Mozafari and Gerdakarch (2012) |
Chandler |
Shoot bud |
MS +KN+BAP+GA3 |
Kaur et al. (2005) |
|
Sweet Charlie |
Runner tips |
MS+TDZ |
Rekha et al. (2012) |
|
camarosa |
Runner tips and Nodal segments |
MS + BAP + NAA |
Tanziman et al. (2012) |
|
Bari |
Tip runner |
MS+BAP |
Ashrafuzzaman et al. (2013) |
|
Camarosa |
Shoot tips |
MS+BAP+TDZ |
Fatemeh et al. (2010) |
|
Senga Sengana, Elsanta |
Crown shoot and tips |
MS+BAP+BA+GA3 |
Litwińczuk et al. (2009) |
|
Chandler Oso-grande Camarosa |
Nodal segment |
MS+BAP+NAA |
Mir et al. (2010) |
|
Iranian cultivar |
Nodal segmnet |
MS+BAP+KN |
Moradi et al. (2011) |
|
AOG, JP-2, JP-3, Camarosa, Sweet Charli, Grant, Mountain, Festival |
Runner tips |
MS+BAP+KN |
Tanziman et al. (2013) |
|
micropropagation via adventitious shoots |
Chandler, Camarosa, Sweet Charlie |
Leaf disc Petiole Stipule Root segment |
MS+BAP+TDZ+2,4-D |
Passey et al. (2003) |
Bounty |
sepal |
MS+TDZ+2,4-D |
Debnath et al. (2005) |
|
Chandler x Totem |
cotyledons |
MS+BAP/NAA |
Miller and Chandler (1990) |
|
JP-2 |
Immature leaves |
MS + BAP + NAA |
Karim et al. (2011) |
|
Iranian cultivars |
Leaf discs |
MS+BAP+NAA |
Mahmoud and Kosar (2013) |
|
Bounty |
Leaf discs, sepal, petiole |
MS+TDZ |
Debnath (2006) |
|
Gorella, Redgault |
Petiole, flower buds |
MS+2,4-D+BAP |
Faucault and Letouze (1987) |
|
Addie, Dana, Gea, Santame |
Stipule, roots |
MS+BAP+IBA |
Rugini and Orlando (1992) |
|
Chandler |
Lefa discs |
MS+TDZ |
Hussaini and Abdin (2007) |
|
Camarosa, Gaviota, Seascape |
Leaf discs |
MS+TDZ |
Mohamed et al. (2007) |
|
Pbgel-2000 |
Nodal segment leaf |
MS+BAP+2,4-D+proline |
Biswas et al. (2007) |
|
Micropropagtion via somatic embryogenesis |
Sweet Charlie chandler |
Leaf disc petiole |
MS +BAP+2,4-D |
Omar et al. (2013) |
Camarosa Paros kurdistan |
Leaf, nodal segment, petiole, stamen |
MS+picloram |
Gerdakaneh and Zohari (2013) |
|
Selva, camarosa |
leaf |
MS+picloram |
Kordestani and Karimi (2008) |
|
camarosa |
Leaf disc |
MS+2,4-D+BA |
Swapnil et al. (2017) |
|
Sweet Charlie |
Petiole segments |
MS+TDZ+2,4-D |
Pallavi et al. (2011) |
|
Chandler |
Leaf discs |
MS + TDZ |
Hussaini and Abdin (2007) |
3. Virus elimination
Vegetative propagation of Fragaria sp. is traditionally carried out using stolons. This system of propagation is slow facilitate the spread of plant diseases, particularly viral pathogens (Mozafari and Gerdakaneh 2012; Quiroz et al. 2017). Many of viruses can greatly reduce yield, rapidly spread in the field without exhibiting evident symptoms (Martin and Tzanetaki 2006). The yield reduction caused by some viruses may be up to 80% (Thompson and Jelkman 2003).In some cases, individual virus strains may not constitute an economic problem but multiple virus infections are reputed to be more severe.
Initially, many of the virus strawberry diseases were described based upon symptoms. More recently, significant progress has been made in the molecular characterization of the aphid-borne viruses, the identification and characterization of several whitefly-borne viruses, and the molecular characterization of viruses associated with several of the “graft transmissible virus-like diseases” of strawberry. Currently, detection methods based on molecular reverse transcription–polymerase chain reaction (RT-PCR) are developed for the most viruses infecting strawberry. Seven aphid-transmitted viruses are reported in strawberry: Strawberry crinkle virus (SCV), Mild yellow edge disease virus (SMYEV), Strawberrymottle virus (SMoV), Strawberry vein banding virus (SVBV), Strawberry pseudo mild yellow edge virus (SPMYEV), Strawberry chlorotic fleck virus (SCFV) and Strawberry latent C virus (SLCV). Among these SCV, SMYEV, SMoV, and SVBV have been considered the four most economically important viruses (Martin and Tzanetakis 2006).
In vitro meristem culture and establishment of micropropagation protocols are important tools for solving the problem of viral infection. In recent years, considerable efforts have been made to develop in vitro propagation of the commercial strawberry in order to produce virus-free plants of high quality (Mozafari and Gerdakaneh 2012; Quiroz et al. 2017). Regeneration of virus-free plants is inversely correlated to the meristem size. It’s recommended to associate this technique with thermotherapy for better results of sanitation. However, when meristem size is less than 1 mm, the majority of viruses may be eliminated from the regenerated plants by meristem culture without thermotherapy.
This means that, more the meristem is smaller, more sanitation rate is high (Isac et al. 2010).
Regenerated healthy plants may serve as a mother germplasm that should strictly protected against virus vectors to ovoid recontaminations. Greenhouses with insectproof films are used for their purpose under strict controls (Martin and Tzanetaki 2006). However, nurseries planted with in vitro plants as mother source are subjected to certification measures that take into account the generation number (F0, F1, F2…) regarding the tolerated rate.
Improved methods of strawberry micropropagtion contributed to the successful eradication of the most important viruses from the frequently commercialized varieties. Those viruses are latent A strawberry virus, strawberry vein-yellowing, strawberry crinckle virus, strawberry mild yellow-edge virus, strawberry mottle virus (Coman and Neculae 1981 cited by Isac et al. 2010).
4. Germplasm improvement
Traditional germplasm improvement is based on the identification of the most suitable genetic resources from either cultivated or wild germplasm. Starting from wild germplasm, progeny from strawberry crosses with F. virginiana glauca as a common parent have shown significant increases in fruit quality and nutritional features. More recently, tissue culture techniques have been exploited to create genetic, to improve the state of health of the planted material and to increase the number of desirable germplasms available to the plant breeder. Cell culture has produced somaclonal variants with crop-improvement potential. Tissue culture techniques, in combination with molecular techniques, have been successfully used to incorporate specific traits through gene transfer (Brown and Thorpe 1995). A number of molecular studies have targeted strawberry for gene cloning, genetic transformation, fingerprinting and mapping for important agronomic traits such as disease resistance and fruit nutritional quality. These achievements are expanding the potential applications of DNA recombinant technology for the transfer and validation of target genes that control important traits (Mazzetti 2013).
4.1. Somaclonal variationand in vitro selection
Somaclonal variation is defined as the “occurrence of variation in plants regenerated from in vitro cultures” (Larkin and Scowcroft 1981). This variation may be spontaneous (accidentally) or induced. Whatever the way of its occurrence, variation may be desirable or not.
In the case of spontaneous variation, no case of desirable characters was reported in micropropagated strawberry. All of them have been considered as morphological abnormalities including leaf variegation, consisting in a narrow white streak in the leaf blade, chlorosis of the leaves, chlorosis of the leaves and growth changes including dwarfs, compact trusses, lack of runner production, and female sterility (Swart et al. 1981; Adel and Sawy 2007). Somaclonal variation has been associated with changes in chromosome number and structure, deamplification and amplification of genes, transposable element activation and alteration in DNA methylation (Phillips et al. 1994).
In the case of induced variations, selection pressure is generally applied at the collagenesis step and uses several factors of induction. This technology has been adopted as a powerful tool in selection program based in the acquired traits by some cell lines when placed under pressure. Some of those traits are obtained by exposing cells to stresses by phytotoxins from pathogens, herbicides, thermic stress and salinity or drought (Chaleff 1983).
Usually, cells subjected to a suitable in vitro selection pressure are able to recover any variant lines that have developed resistance or tolerance to the stress followed by regeneration of plants from the selected cell. Somaclonal variants can be distinguished by their morphological, biochemical, physiological and genetic characteristics. Molecular markers are powerful tools in genetic identification of somaclonal variation with greater precision than phenotypic and karyologic analysis. Actually, there are various methods available which can be used to detect and monitor tissue culture-derived plants and varietal identification. ISSR (Inter Simple Sequence Repeats) and RAPD (Random Amplified Polymorphic DNA) are the molecular technique for identification of genetic variation (Palei et al. 2015). Strawberries (Fragaria x ananassa Duch.) have been extensively analyzed using randomly generated markers for clone identification and diversity studies. Polymorphism also appears in acid-phosphatase, glutamate-dehydrogenase and peroxidase of regenerated plants (Adel and Sawy, 2007).
In strawberry, research was done to obtain plants resistant/tolerant to biotic stress such as Alternaria alternata (Takahashi et al. 1992), Botritis cinerae (Orlando et al. 1997), Colletotrichum acutatum (Hammerschlag et al. 2006), Fusarium oxysporum (Toyoda et al. 1991), Phytophtora cactorum (Sowik et al. 2001), Rhizoctonia fragaria (Orlando et al. 1997), Verticillium dahlia (Sowik et al. 2003;Zebrowska 2010) and to abiotic stresslike salinity (Dziadczyk et al. 2003) and cold (Rugienius and Stanys 2001). Regeneration of resistant plants is genotype-dependant (Hammerschlag et al. 2006).
4.2. Genetic transformation
Genetic engineering of Fragaria has made notable progress (James et al. 1990; Nehra et al. 1990).The first work has been focused on transformation using genes for pest and herbicide resistance, cold tolerance and ripening (Hussaini and Srivastava 2006; Debnath and Da Silva, 2007).
Transformation was generally achieved viaAgrobacterium tumefaciens-mediated technology carrying the plasmid pBI121 (Ricardo et al. 2003; Folta et al. 2006). Transgenic nature of the shoots was confirmed by the GUS assay and PCR analysis.
Previous studies on strawberry have clearly demonstrated the importance of various factors such as genotype (Liu and standford 1988), type and source of explant (Nehra et al. 1989), hormonal balance and incubation conditions (Liu and Sanford 1988) on efficiency of transformation.
James et al. (1990) found that petioles of ‘rapella’ cultivation were transformed more efficiently than leaf discs. By contrast, the latter explant gave better results than stipules in F. vesca (Alsheikh et al. 2002). Matthews et al. (1998) observed highest transformation rates when using meristematic sections obtained from the base of in vitro proliferating plantlets, but a high percentage of regenerated plants were chimeras.
According to Khammuang et al. (2005), the optimal condition for regeneration of transformed shoots from leaf explants cv. Tiogar was MS medium containing BA (0.1mg/l) and 2,4-D (0.2 mg/l).
A first example concerns recombinant gene encoding antifreeze protein isoform HPLC 6 was successfully constructed using codons which were optimally expressed in the strawberry plant. The antifreeze protein genes, naturally in plasmid pSW1 and modified in plasmid pBB, were introduced instrawberry leaf explants by Agrobacterium tumefaciens LBA 4404. Polymerase chain reaction (PCR) indicated that the transgenes were integrated in the genome of transformants (Khammuang et al. 2005).
The second example concerning the gene encoding the taste-modifying protein miraculin (Toshiyuki et al. 2008). Miraculin was detected in leaves and fruits of the transgenic plants with variable levels ranged from 0.5 to 1 mg/g miracle fruit. High levels of miraculin accumulation were detected in the mature fruits. The transgenic lines were subsequently propagated via runners for three vegetative generations, and miraculin was detected at equal levels in the leaves and fruits of the plants from each generation (Toshiyuki et al.2008).
5. Conclusion
In vitro propagation of strawberry finds its main practical application in mass propagation of virus-free plants. In association with breeding programs micropropagtion may have a significant impact, such as mass production of elite selections, accelerating the breeding process by in vitro selection and germplasm conservation. Meristem tip culture alone or combined with thermotherapy has been utilized to eliminate viruses from infected strawberries (Graham 2005). True-to-type propagules and genetic stability are prerequisites for the application of micropropagtion. In vitro organogenesis and somatic embryogenesis are alternative means of mass scale propagation. The development of somatic embryogenesis of strawberry has not progressed as far as other methods of regeneration. Further studies are then required for optimization of the culture conditions for the induction, development and germination of somatic embryos. Clonal fidelity of micropropagated plants can be monitored at morphological, biochemical, physiological and molecular levels. Molecular markers like ISSR and RAPD are powerful tools in genetic identification of somaclonal variation. In vitro selection, considered as an alternative to genetic transformation, may offer to plant breeders a new approach for strawberry improvement.
In vitro techniques are important tools for modern plant improvement programs to introduce new traits into selected plants and to multiply elite selections. Used in conjunction with classical breeding methods, an efficient in vitro shoot proliferation and regeneration system could accelerate cultivar development programs. Standardization of an efficient regeneration system for each strawberry genotype is generally a necessary pre-requisite for the successful development of transgenic plants.
6. Références
Adel EL and Sawy M (2007) Somaclonal variation in micro-propagated strawberry detected at the molecular level. International Journal of Agriculture & Biology. 9: 72–725
Alsheikh MK, Suso HP, Robson M, Battey NH, Wetten A (2002) Appropriate choice of antibiotic and Agrobacterium strain improves transformation of antibiotic sensitive Fragaria Vesca semperfloreus. Plant Cell Rep 20:1173-1180
Ashrafuzzaman M, Faisal SM, Yadav D, Khaman D, Rayhan F (2013) Micropropagation of strawberry (Fragaria x ananassa) through runner culture. Bangladesh J Agri Res. 38:467-472
Barceló M, El-Mansouri I, Mercado JA, Quesada MA, Alfaro FP (1998) Regeneration and transformation via Agrobacterium tumefaciens of the strawberry cultivars chandler. Plant Cell Tissue Organ Culture. 54: 29-36.
Biswas MK, Islam R, Hossain M (2008) Micro propagation and field evaluation of strawberry in Bangladesh. J Agric Technol 4: 167-182.
Biswas MK, Hossain M, Ahmed MB, Roy UK, Karim R, Razvy MA, Salahin M, Islam R (2007) Multiple shoots regeneration of strawberry under various colour illuminations. American-Eurasian Journal of Scientific Research 2: 133-135.
Boxus Ph (1999) Micropropagation of strawberry via axillary shoot proliferation. In: RD Hall (ed) Methods in molecular biology. Plant Cell Culture Protocols. Humame Press Inc. Totona NJ. 11:103-114
Boxus (1974)The production of strawberry plants by in vitro micropropagtion. J Hort Sci 49:209-210
Boxus (1992) Mass production of strawberry and new alternative for some horticultural crops. In: Kurata K, Kozai T (eds). Transplant production systems. Kluwer Academic Publishers. The Netherlands. Pp:151-162
Brown DC, Thorpe TA (1995)Crop improvement through tissue culture.World J Microbiol Biotechnol 11:409-15
Cao X, Hammershlag FA (2000) Improved shoot organogenesis from leaf explants of highbush blueberry. Hortscience 35:945-947
Cerović R, Ružić D (1989) Micropropagation of strawberry cvs. Cacanska Rana and Senga Sengana, pomological-biochemical characteristics of micropropagated plants. Acta Hortic 265:353-358
Chaleff RS (1983) Isolation of agronomically useful mutants from plant cell cultures. Science 219:676-682
Darnell R, Cantliffe D, Kirschbaum D, Chandler C (2003) In: Janick J (ed) The physiology of flowering in strawberry. Hortic Dev 28:325-349
Debnath SC (2003) Micropropagtion of small fruits. In: Jain SM, Ishii K (eds). Micropropagation of woody trees and fruits. Kluwer Academic Publishers. Dordrecht, Germany. pp: 465-506
Debnath SC (2005) Strawberry sepal: Another explants for thidiazuron-induced adventitious shoot regeneration. In vitro Cell Dev Biol-pl. 41:671-676
Debnath SC (2006) Zeatin overcomes thidiazuron_induced inhibition of shoot elongation and promotes rooting in strawberry culture in vitro. J hort Sci biotech 81:349-354
Debnath SC and Da Silva JAT (2007) Strawberry culture in vitro : Applivations in genetic transformation and biotechnology. Fruit Vegetable Cereal Science Biotechnology 1 :1-12
Donnoli R, Sunseri F, Martelli G, Greco I (2001) Somatic embryogenesis and genetic transformation in Fragaria spp. Acta Hortic 560:236-240
Dziadczyk P, Bolibok H, Tyrka M, Hortijuski JA (2003) In vitro selection of strawberry (Fragaria x ananassa) clones tolerant to salt stress. Euphytica 132:49-55
El Mansouri I, Mercado JA, Valpuesta V, Lopez-Aranda JM, Pliego-Alfaro F, Quesada MA (1996) Shoot regeneration and Agrobacterium-mediated transformation of Fragaria vesca L. Plant Cell Rep 15:642-646
Fatemeh H, Mehran AA, Ghizan S, Azmi AR, Hussain K (2010) Micropropagation of strawberry cultivar camarosa: prolific shoot regeneration from in vitro shoot tips using thidiazuron with N6-benzylamino-purine. Hortscience 45:453-456
Fehér A, Pasternak TP, Dudits D (2003) Transition of somatic plant cells to an embryogenic state Plant Cell Tissue Organ Cult. 74:201–28.
Folta KM, Howard L, Dhingra A, Stewart PJ, Chandler CK (2006) Characterization of LF9, an octoploid strawberry mesophyll cells. 86th annual meeting of the American society of horticultural science 25:569-570
Foucault C, Letouze R (1987) In vitro régénération de plantes de fraisier à partir de fragments de petiole et de bourgeons floraux. Biol Plant 29:409-414
George EF, Hall MA, De Klerk GI (2008) Plant tissue procedure-background. In: George EF, Hall MA, De Klerk GI (eds) Plant propagation by tissue culture. Springer Netherlands. pp: 1-28
Gerdakaneh M, Zohari M (2013) The effect of picloram on somatic embryogenesis of different explants of strawberry (Fragaria ananassa Duch.). Brit Biotech J. 3:133-142
Graham J (2005) Fragaria strawberry. In: Litz R (ed) Biotechnology of fruit and nut crop. Biotechnology in Agriculture Series No 29, CAB International. Wallingford, UK. pp: 456-474
Haccius B (1978) Question of unicellular origin of non-zygotic embryos in callus cultures. Phyto morphology 28:74-80
Hammerschlag F, Garces S, Koch-Dean M, Ray S, Lewers K, Maas J, Smith BJ (2006)In vitro response of strawberry cultivars and regenerants to Colletotrichum acutatum. Plant Cell Tiss Org Cult 84:255–261.
Hanhineva K, Kokko H, Kärenlampi S (2005) Shoot regeneration from leaf explants of five strawberry (Fragaria × ananassa) cultivars in temporary immersion bioreactor system. In Vitro Cell Dev Biol Plant 41:826–31.
Hannum SM (2004) Potential impact of strawberries on human health: a review of the science. Ctri Rev Food Sci Nut 44:1-17
Heinonen MI, Meyer AS, Frankle EN (1998) Antioxidant activity of berry phenolics on human low-density lipoprotein and liposomeoxidation. J Agric Food Chem 46:4107-4112
Huetteman CA, Preece JE (1993) Thidiazuron: A potent cytokinin for woody plant tissue culture. Plant Cell Tiss Org Cult 33:105-119
Hussaini AM, Abdin MZ (2007) Interaction effect of light, temperature and TDZ on the regeneration potential of leaf discs of Fragaria x ananassa Duch. In Vitro Cell Dev Biol-Pl 43:576-580
Hussaini AM, Srivastava DK (2006) Genetic transformation in strawberry. Asian Microb Biotech Environ Sci 8:75-81
Hussaini AM, Aquil S, Bhat M, Qadri T (2008) High efficiency of direct somatic embryogenesis system for strawberry (Fragaria x ananassa) cultivar Chandler. J Crop Sci Biotechnol 11:107-110
Isac V, Coman T, Marinescu L, Isac M, Teodorescu A, Popescu A, Coman M Plopa C (2010) Achievements and trends in the use of tissue culture for the mass propagation of fruit plants and germplasm preservation at the research institute for fruit growing, Pitesti, Romania. Rom Biotech Let 15:92-101
James DJ, Passey AJ, Barbara DJ (1990) Agrobacterium-mediated transformation of the cultivated strawberry (Fragaria x ananassa Duch.) using disarmed binary vectors. Plant sci 69:79-94
Jemmali A, Boxus Ph, Kinet JM (1992) Are strawberry arising from adventitious stipule buds tue-to-type ? Acta Hortic 319 :171-176
Jemmali A, Boxus Ph, Dekegel D, Van Heule G (1994) Occurrence of spontaneous shoot regeneration on leaf stipules in relation to hyperflowering response in micropropagation strawberry plantlets. In vitro cell Dev Biol Pl 30 :192-195
Jimenez M, Bangerth F (2001) Endogeneous hormones levels in explants and in embryogenic and non-embryogenic cultures of carrot. Physiol Plant 111 :389-392
Jones OP, Waller BJ, Beech MG (1988) The production of strawberry plants from callus culture. Plant Cell Tiss Org Cult 12:751-759
Karhu S, Hakala K (2002) Micropropagation of strawberry on te field. Acta Hortic 2:182
Karim MR, Abdul Aziz M, Roy UK, Hoque MA, Hossain MM (2011) In vitro response of strawberry (Fragaria x ananassa) for callus induction and shoot regeneration. Int J Agr R 1:29-36
Kaur R, Hernaut G, Sharma DR (2005) Low cost strategy for micropropagtion of strawberry (Fragaria x ananassa Duch.) cv. Chandler. Acta Hortic 696 :129-133
Khammuang S, Hanmuangjai P, Won-groung S (2005) Agrobacterium-mediated transformation of modified anti freeze protein gene in strawberry. J Sci Technol 17 :1302-1304
Kordestani G, Karami O (2008) Picloram-Induced Somatic Embryogenesis in Leaves of Strawberry (Fragaria ananassa L.). Acta Biologica Cracoviensia Series Botanic. 50:69–72
Kumar N, Reddy MP (2011) In vitro plant propagation. J Forest Sci. 27:61-72
Landi L, Mezetti B (2006) TDZ, an auxin and genotype effects on leaf organogenesis in Fragaria. Plant Cell Rep 25:281-288
Larkin PJ, Scowcroft WR (1981) Somaclonal variation- a noval source of variability from cell cultures for plant improvement. Theor and Appl Gen 60:197-214
Larson RA (1988) The antioxidant of higher plants. Phytochem 27:969-978
Laux T Jugens G. (1997) Embryogenesis: new start in life. The Plant Cell. 9:989–1000.
Lis EK (1993) Strawberry plant regeneration by organogenesis from peduncle and stolon segments. Acta Hort 708:253-254
Litwińczuk W, Zubel A (2005) Growth in vitro cultures of strawberry (fragaria w ananassa) depending on different phtoperiod. Folia Hort 17:81-87
Litwińczuk W, Okotokiewiecz E, Matyaszek I (2009) Development of in vitro shoot cultures of strawberry (Fragaria anananassa Duch.) ‘Senga sengana’ and ‘Elsanta’ under influence of high doses of gibberellic acid. Folia Hort 21:43-52
Litz RE, Gray DJ (1995) Somatic embryogenesis for agricultural improvement. World J Microbiol Biotechnol. 1:416–425
Liu ZR, Sanford JC (1988) Plant regeneration by organogenesis from strawberry leaf and runner cultur. Hortscience 23;1056-1059
Martin RR, Tzanetakis IE (2006). Characterization and recent advances in detection of strawberry viruses. Plant Dis. 90:384–396.
Matthews H, Dewey V, Wagner W, Bestwick RK (1998) Molecular and cellular evidence of chimaeric tissues in primary transgenics and elimination of chimaerism through improved selection protocols. Trans Res 7:123-129
Mazzetti B (2013) Breeding and biotechnology for improving the nutritional quality of strawberry. J Berry Res 3:127-133
Merkle SA, Parrott WA, Flin BS (1995) Morphogenic aspect of somatic embryogenesis. In: Torpedo (ed) In vitro embryogenesis in plant. Klauwer Academic Publishers. Netherlands. pp:155– 203
Miller AR, Chandler CK (1990) Plant regeneration from excised cotyledon of mature strawberry achenes. Hortscience 25:569-571
Mir JI, Ahmed N, Rashid R, Wani SH, Mir H, Sheikh MA (2010) Micropropagation of strawberry (Fragaria x ananassa). Crop improvement. 37:153-156
Mohamed FM, Beltagi MS, Ismail MA, Omar GF (2007) High frequency, direct shoot regeneration from greenhouse derived leaf discs of six strawberry cultivars. Pak J Bio Sci 10:96-101
Mohan R, Chui EA, Biais LA, Soccol CR (2005) Alternative in vitro propagation: use of sugarcane bagasse as a low cost support material during rooting stage of strawberry cv. Dover. Braz Arch Biol Tech 48:37-42
Moradi K, Otroshy M, Azimi MR (2011) Micropropagation of strawberry by multiple shoot regeneration tissue cultures. J Agri Tech. 7:1755-1763
Mozafari AA, Gerdakaneh M (2012) Influence of media and growth regulators on regeneration and morphological characteristics of strawberry cvs. Kurdistan and Merck (Fragaria x ananassa Duch.) Int J Pl Physiol Biochem 4:99-104
Murashige T, Skoog F (1962)A revised medium for rapid growth and bioassays with tobacco tissues cultures. Physiol Plant 15:473-479
Nehra NS, Stushnoff C, Kartha KK (1989) Direct shoot regeneration form strawberry leaf disks. J Am Soc Hortic Sci 114:1014-1018
Nehra NS, Chibbar RN, Kartha KK, Dalta RSS, Crosby WL, Stushnoff C (1990) Genetic transformation of strawberry by GArobacterium-tumefasciens using a leaf disk regeneration system. Plant Cell Rep 9:293-298
Nehra NS, Kartha KK (1994) Meristem and Shoot tip Culture: Requirements and Applications. In: Vasil IK and Thorpe TA (eds) Plant Cell and Tissue Culture. Kluwer Academic Publishers, Netherlands. pp: 37-71.
Omar GF, Mohamed FH, Haensh KT, Sarg SH, Morsy MM (2013) Somatic embryo-like structures of strawberry regenerated in vitro on medium supplemented with 2,4-D and BAP. Ind J Exp Biol. 51:739-745
Orlando R, Magro P, Rugini E (1997) Pectic enzymes as a selective pressure tool for in vitro recovery of strawberry plants with fungal disease resistance. Plant Cell Rep 16:272-276
Palei S, Das AK, Rout GR (2015) In vitro studies of strawberry – An important fruit crop. J Pl Sci Res 31:115-131
Pallavi CM, Rekha R, Neelambika TM (2011) Indirect somatic embryogenesis from petiole segment in strawberry cv. Sweet Charlie. Ind J Hortic 68:24-30
Passey AJ, Barrett KJ, James DJ (2003) Adventitious shoot regeneration from seven commercial strawberry cultivars (Fragaria x ananassa Duch.) using a range of explant types. Plant Cell Replic. 21:397-401
Phillips RL, Kaeppler SM, Olhoft P (1994) Genetic instability of plant tissue cultures:breakdown of normal controls. Proc Nat Ac Sci 91:5222-5226
Popescu AN, Isac VS, Coman MS, Radulescu MS (1997) Somaclonal variation in plants regenerated by organogenesis from callus culture of strawberry (Fragaria x ananassa). Acta Hortic. 439: 89-95.
Qin Y, Zhang S, Zhang LX, Zhu D, Asghar S (2005) Response of strawberry cv. Toyonoka in vitro to silver nitrate (AgNO3). Hortscience 40:747-751
Quesada MA, Martin-Pizarro C, Garcia-Cago JA, Posé s, Santiago N, Sesmero R, Pliego-Alfaro F, Mercado JA (2007) Transgenic dtrawberry: Current status and future perspectives. Transg Plant J 1:280-288
Quiroz KA, Berrios M, Carrasco B, Retamales JB, Caligari PDS, Garcia-Gonzales R (2017) Mersitem culture and subsequent micropropagtion of Chilean strawberry –Fragaria chiloensis (L.) Duch.). Biol Res 50:20-31
Quiroz-Figueroa FR, Rojas-Herrera R, Galaz-Avalos RM, Loyola-Vargas VM (2006) Embryo production through somatic embryogenesis can be used to study cell differentiation in plants. Plant Cell Tiss Organ Cult. 86:285–291.
Raemakers CJJM, Joedsen E, Visser RGFL (1995) Secondary somatic embryogenesis and applications in plant bredding. Euphytica 81 :93-98
Rekha A, Pallavi M, Meti N (2012) Micropropagation of strawberry cultivar Sweet charlie through axillary shoot proliferation. J App Hort 14 :71-73
Ricardo YG, Coll Y, Castagnaro A, Diaz Ricci JC (2003) Transformation of strawberry cultivar using a modified regeneration medium. Hortscience 38:227-280
Rugienius R, Stanys V (2001) In vitro screening of strawberry plants for cold resistance. Euphytica 122:169-277
Rugini E, Orlando R (1992) High efficiency shoot regeneration from calluses of strawberry (Fragaria ananassa Duch.) stipules of in vitro cultures. J Hortic Sci 67:577-582.
Schween G, Schwenkel HG (2003) Effect of genotype on callus induction shoot regeneration, and phenotypic stability of regenerated plants in greenhouse of Primula ssp. Plant Cell Org Tii Cult 72:53-61.
Shulaev V, Korban SS, Sosinski B, Abbott AG, Aldwinkle HS, Folta KM, Lezzoni A, Main D, Arus P (2008) Multiple models for Rosaceae genomics. Plant physiol 147:985-1003
Sorvari S, Ulvinen S, Hietarant T, Hiirsalmi H (1993) Preculture medium promotes direct shoot regeneration from micropropagated strawberry leaf disks. Hortscience 662:227-280
Sowik I, Bielenin A, Michalczuk L (2001) In vitro testing of strawberry resistance to Verticillium dahlia and Phytophtora cactorum. Sci Hortic. 88:31-40
Sowik I, Wawrzynczak D, Michalczuk L (2003) Ex vitro establishment and greenhouse performance of somaclonal variants of strawberry selected for resistance to Verticillium dahlia. Acta Hortic. 616:497-500.
Stasolla C, Yeung EC (2003) Recent advances in conifer somatic embryogenesis: improving somatic embryo quality. Plant Cell Tissue Organ Cult. 74:15–35.
Steward FC, Mapes MO, Meats K (1958) Growth and organized development of cultured cells, II. Organization in cultured grown from freely suspended cells. Am J Bot 45:704-708
Swapnil GW, Komal RP, Ramling HT, Abhijeet SJ (2017) Somatic embryogenesis in strawberry (Fragaria x ananassa) var. camarosa. GJBB. 6: 309-313.
Swartz HJ, Galletta Gj, Zimmerman RH (1981) Field performance and phenotypic stability of tissue culture-propagated strawberries. J Am Soc Hortic Sci 106:667-673
Takahashi H, Takatsugu T, Tsutomu (1992) Resistance plants to Alternaria alternate strawberry pathotype selected from ca liclones of strawberry cultivar Morioka-16 and their characteristics. J Jpn Soc Hortic Sci 61:323-329.
Tanziman A, Karim R, Karim MR, Ahmed S, Island R, Hossain M (2012) Effects of different hormones on in vitro regeneration of strawberry (Fragaria x ananassa Duch.). Int J Biosci. 2:86-92
Thompson JR, Jelkman W (2003) The detection and variation of strawberry mottle virus. Plant Dis 87: 385- 390.
Tian M, Gu Q, Zhu M (2003) The involvement of hydrogen peroxide and antioxidant enzymz in the process of shoot organogenesis of strawberry callus. Plant Sc 165 :701-707
Titov S, Bhowmik SK, Mandal A, Alan MS, Nasiruddin S (2006) Control of phenolic compound secretion and effect of growth regulators for organ formation from Musa spp. (cv. Kanthali) floral bud explants. Am J Bioch Biotech 2 :97-104
Torné JM, Moysset L, Claparols I, Simon E (1996) Photocontrol of somatic embryogenesis and polyamine content in Aranjia sericifera petals. Physiol Plant 89 :413-418
Toshiyuki S, Megumu Y, Hyeon-Jin S, Tadayoshi H, Hiroshi E (2008) Transgenic strawberry expressing the taste-modifying protein miraculin. Plant Biotech 25 :329–333
Toyoda H, Horikoshi K, Yamano Y, Ouchi S (1991) selection of Fusarium wilt disease resistance from regenerants derived from callus of strawberry. Plan Cell Rep 10:167-170
Wang H, Cao G, Prior RL (1996) Total antioxidant capacity of fruits. J Agri Food Chem 44:701-705
Wang SY, Jiao H (2000) Scavenging capacity of berry crops on superoxide radicals, hydrogen peroxide, hydroxyl radicals, and singlet oxygen. J Agri Food Chem 48:5677-5684
Wang YH, Bhalla PL (2004) Somatic embryogenesis from leaf explants of Australian fan flower (Scaevola aemula R. Br.) Plant Cell Rep. 22:408–14
Williams EG, Maheswaran G (1986) Somatic embryogenesis: factors influencing coordinated behavior of cells as an embryogenic group. Ann Bot 57:443-448
Zebrowska JI (2010) In vitro selection breeding of strawberry (Fragaria x ananassa Duch.). Commun Agric Appl Biol Sci 75 :699-704
Zebrowska JI, Czernas J, Gawronski J, Hortynski JA (2003) Suitable of strawberry (Fragaria x ananassa Duch.) microplants to the field cultivation. Food Agri Env 1:190-193
Zhao Y, Liu Q, Davis RE (2004) Transgene expression in strawberries driven by heterologous phloemspecific promoter. Plant Cell Rep. 23:224–230.
Zimmerman RH (1981) Micropropagtion of fruit plants. Acta Hort 120:217-222