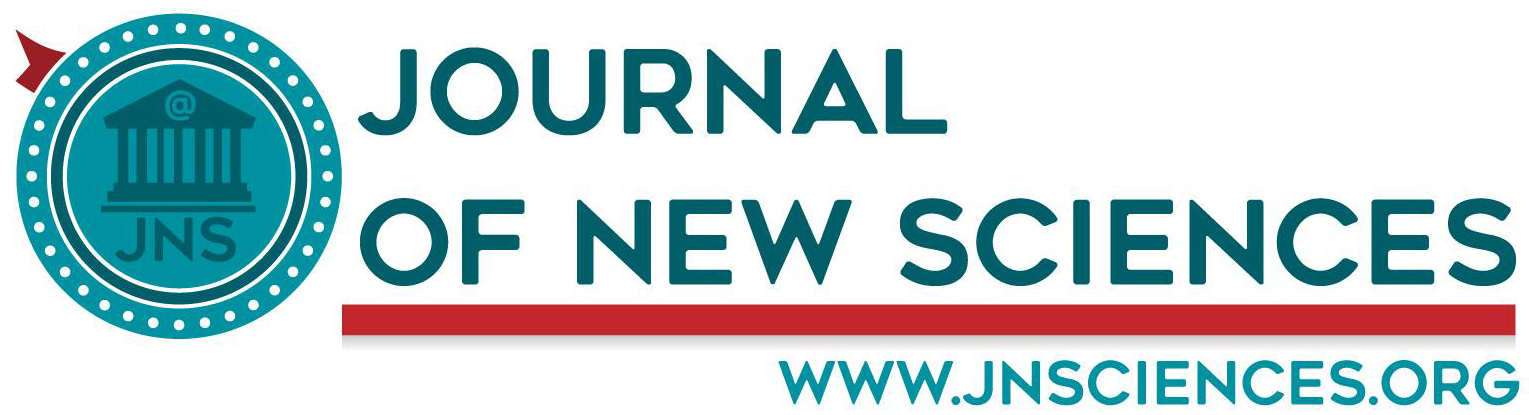
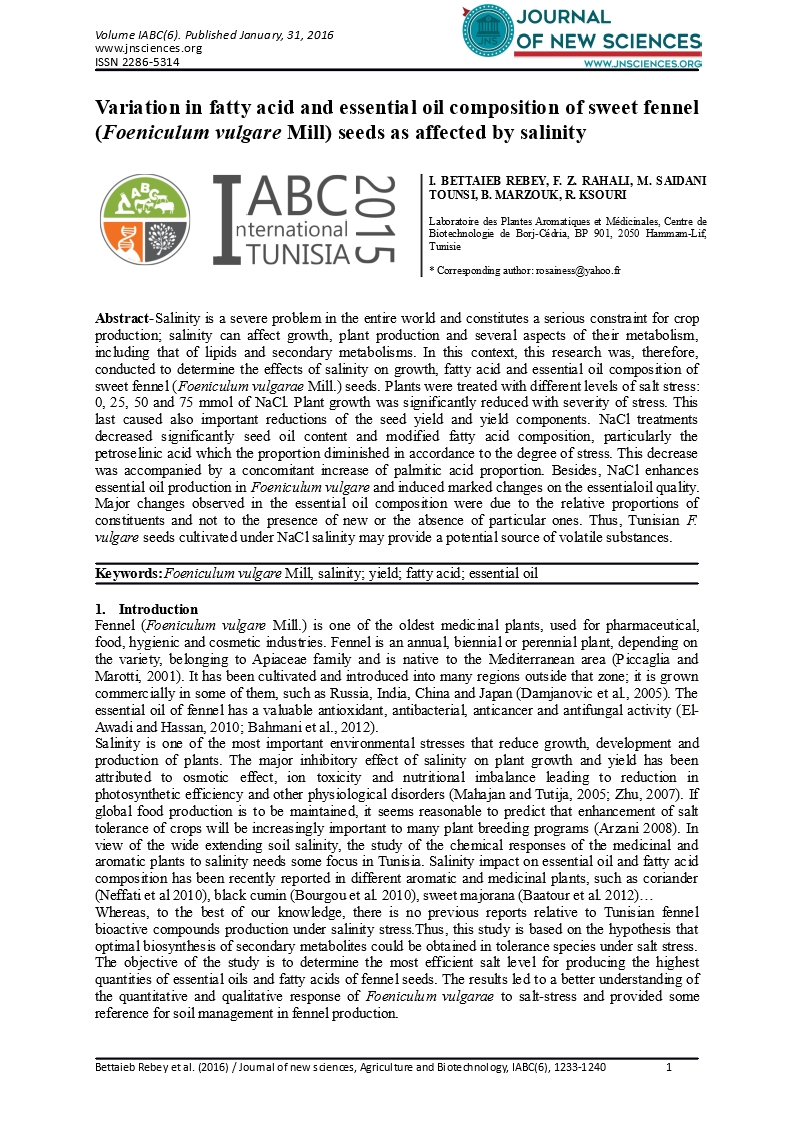
- Category: Volume Spécial (Conférence IABC 2015)
- Hits: 8497
Variation in fatty acid and essential oil composition of sweet fennel (Foeniculum vulgare Mill) seeds as affected by salinity
I. BETTAIEB REBEY
F. Z. RAHALI
M. SAIDANI TOUNSI
B. MARZOUK
R. KSOURI
Laboratoire des Plantes Aromatiques et Médicinales, Centre de Biotechnologie de Borj-Cédria, BP 901, 2050 Hammam-Lif, Tunisie
Abstract-Salinity is a severe problem in the entire world and constitutes a serious constraint for crop production; salinity can affect growth, plant production and several aspects of their metabolism, including that of lipids and secondary metabolisms. In this context, this research was, therefore, conducted to determine the effects of salinity on growth, fatty acid and essential oil composition of sweet fennel (Foeniculum vulgarae Mill.) seeds. Plants were treated with different levels of salt stress: 0, 25, 50 and 75 mmol of NaCl. Plant growth was significantly reduced with severity of stress. This last caused also important reductions of the seed yield and yield components. NaCl treatments decreased significantly seed oil content and modified fatty acid composition, particularly the petroselinic acid which the proportion diminished in accordance to the degree of stress. This decrease was accompanied by a concomitant increase of palmitic acid proportion. Besides, NaCl enhances essential oil production in Foeniculum vulgare and induced marked changes on the essentialoil quality. Major changes observed in the essential oil composition were due to the relative proportions of constituents and not to the presence of new or the absence of particular ones. Thus, Tunisian F. vulgare seeds cultivated under NaCl salinity may provide a potential source of volatile substances.
Keywords: Foeniculum vulgare Mill, salinity; yield; fatty acid; essential oil
-
Introduction
Fennel (Foeniculum vulgare Mill.) is one of the oldest medicinal plants, used for pharmaceutical, food, hygienic and cosmetic industries. Fennel is an annual, biennial or perennial plant, depending on the variety, belonging to Apiaceae family and is native to the Mediterranean area (Piccaglia and Marotti, 2001). It has been cultivated and introduced into many regions outside that zone; it is grown commercially in some of them, such as Russia, India, China and Japan (Damjanovic et al., 2005). The essential oil of fennel has a valuable antioxidant, antibacterial, anticancer and antifungal activity (El-Awadi and Hassan, 2010; Bahmani et al., 2012).
Salinity is one of the most important environmental stresses that reduce growth, development and production of plants. The major inhibitory effect of salinity on plant growth and yield has been attributed to osmotic effect, ion toxicity and nutritional imbalance leading to reduction in photosynthetic efficiency and other physiological disorders (Mahajan and Tutija, 2005; Zhu, 2007). If global food production is to be maintained, it seems reasonable to predict that enhancement of salt tolerance of crops will be increasingly important to many plant breeding programs (Arzani 2008). In view of the wide extending soil salinity, the study of the chemical responses of the medicinal and aromatic plants to salinity needs some focus in Tunisia. Salinity impact on essential oil and fatty acid composition has been recently reported in different aromatic and medicinal plants, such as coriander (Neffati et al 2010), black cumin (Bourgou et al. 2010), sweet majorana (Baatour et al. 2012)…
Whereas, to the best of our knowledge, there is no previous reports relative to Tunisian fennel bioactive compounds production under salinity stress.Thus, this study is based on the hypothesis that optimal biosynthesis of secondary metabolites could be obtained in tolerance species under salt stress. The objective of the study is to determine the most efficient salt level for producing the highest quantities of essential oils and fatty acids of fennel seeds. The results led to a better understanding of the quantitative and qualitative response of Foeniculum vulgarae to salt-stress and provided some reference for soil management in fennel production.
-
Materials and methods
-
Plant material and growth conditions
Fennel seeds were collected from cultivated plants in the region of Menzel Temime (Northeastern Tunisia). Botanical identification of this species was carried out by Prof. A. Smaoui (Biotechnologic Center in Borj-Cedria Technopark, Tunisia), and a voucher specimen has been kept in our laboratory for future reference. Seeds were germinated on an inert substrate at 25 °C. After 10 days, seedlings were transferred to 6-l plastic pots (6 plants per pot) and were hydroponically cultivated, using aerated quarter strength Hoagland’s solution, containing macronutrients (mM): MgSO4 (0.5), KH2PO4 (0.25), KNO3 (1.25), Ca (NO3)2 (1.25). The medium also contained iron as complex EDTA–K–Fe and micronutrients as a mixture of salts: MnCl2, CuSO4_5H2O, ZnSO4_7H2O, Mo7O24 (NH4)6_4H2O and H3BO3 (Hoagland and Arnon 1950). Two leaf seedlings were separated in four groups irrigated with a nutrient solution supplemented with different NaCl concentrations (0, 25, 50 and 75 mM). To avoid osmotic shock, salt concentrations increased stepwise daily with 25 mM NaCl. The nutrient solutions were replaced each 4 days. The experiment was performed under controlled conditions (18–25 °C temperature, 16/8 h light/darkness, 60–80% relative humidity and under artificial light of 141 µmol/m/s (6000 lx)). Seeds were harvested at the fruiting stage, 15 weeks after treatment and weighted.
-
Oil extraction
Fennel seeds were finely ground in an electric grinder (IKA-WERK. Type: A: 10). Ten grams of each ground sample were extracted using a soxhlet-apparatus with 100 ml hexane (Analytical Reagent, LabScan, Ltd., Dublin, Ireland) for 6h. The extraction was protected against light. Oil was removed after mixture filtration and solvent evaporation under reduced pressure.
-
Total lipid extraction
Total lipids from seeds were extracted by the modified method of Bligh and Dyer (1959), according to Marzouk and Cherif (1981). Thus, 0.5 g air-dried seed were fixed in boiling water for 5 min and then ground manually with chloroform/methanol/hexane mixture (1:2:1, v/v/v). After washing with water of fixation and decantation during 24 h at + 4 °C, the organic phase containing total lipids was dried under a stream of nitrogen, dissolved in toluene-ethanol (4:1, v/v) and stored at -20 °C for further analyses.
-
Fatty acid methylation and analysis
Total fatty acids were converted into their methyl esters using 3% sodium methylate in methanol according to the method described by Cecchi et al. (1985). Heptadecanoïc acid (C17:0) methyl ester was used as an internal standard in order to quantify fatty acids. The superior phase that contains fatty acid methyl esters (FAMEs) was aspired and the solvent volume reduced under a stream of nitrogen, prior to analysis. FAMEs were analysed by gas chromatography using a Hewlett-Packard 6890 chromatograph (Agilent Technologies, Palo Alto, CA, USA) equipped with a flame-ionization detector (FID) and an electronic pressure control (EPC) injector. They were separated on a RT-2560 capillary column (100 m length, 0.25 mm i.d., 0.20 mm film thickness). The oven temperature was kept at 170 °C for 2 min, followed by a 3 °C min−1 ramp to 240 °C and finally held there for an additional 15 min period. Nitrogen (U) was used as carrier gas at a flow rate of 1.2 mL min−1. The injector and detector temperatures were maintained at 225 °C. A comparison of the retention times of the FAMEs with those of co-injected authentic standards (Analytical Reagent, LabScan, Ltd., Dublin, Ireland) was made to facilitate identification.
-
Essential oil extraction
Samples of air-dried fennel seeds were finely ground in an electric grinder (IKA-WERK. Type: A:10). Fifty grams of each ground material were subjected to hydrodistillation for 120 min. The hydrodistillation was performed by a simple laboratory Quik-fit apparatus which consisted of a 1000 mL steam generator flask, a distillation flask, a condenser and a receiving vessel. The distillate obtained was extracted using diethyl-ether as solvent (v/v) and dried over anhydrous sodium sulphate. The organic layer was then concentrated at 35 °C using a Vigreux column and the essential oil stored at −20 °C prior to analysis.
-
Gas Chromatography (GC-FID)
Analytical gas chromatography was carried out on a Hewlett-Packard 6890 gas chromatograph equipped with a flame ionization detector (FID) and an electronic pressure control (EPC) injector. A polar HP Innowax (PEG) column and an apolar HP-5 column (30 m x 0.25 mm, 0.25 µm film thickness) were used. The flow of the carrier gas (N2) was 1.6 mL min-1. The split ratio was 60:1. The analysis was performed using the following temperature program: oven temps isotherm at 35 °C for 10 min, from 35 to 205 °C at the rate of 3 °C min-1 and isotherm at 205 °C during 10 min. Injector and detector temperature were held, respectively, at 250 and 300 °C.
-
Gas Chromatography-Mass Spectrometry (GC-MS)
GC-MS analysis was performed on a gas chromatograph HP 5890 (II) interfaced with a HP 5972 mass spectrometer with electron impact ionization (70 eV). A HP-5MS capillary column (30 m x 0.25 mm, 0.25 µm film thickness) was used. The column temperature was programmed to rise from 50°C to 240 °C at a rate of 5°C min-1. The carrier gas was helium with a flow rate of 1.2 mL min-1; split ratio was 60:1. Scan time and mass range were 1s and 40-300 m/z, respectively.
-
Compounds identification
Compounds identification was assigned by comparison of their retention indices relative to (C8–C22) n-alkanes with those of literature or with those of authentic compounds available in our laboratory. Further identification was made by matching their recorded mass spectra with those stored in the Wiley/NBS mass spectral library of the GC–MS data system and other published mass spectra (Adams 2001).The percentage determination was based on peak area normalization without using correction factors.
-
Statistical analysis
Data were subjected to statistical analysis using statistical program package STATISTICA.Percentage of each parameter was the mean of six replicates ± S.D and the differences between individual means were deemed to be significant at p<0.05.
-
Results and discussion
-
Effect of salinity on plant growth and yield components
The results for the effects of salt treatment on plant growth and yield components are presented in Table 1. As can be seen, the addition of 25 and 50 Mm NaCl to the culture of F. vulgare provoked a light drop of the plant height, whereas 75Mm NaCl diminished plant height by about 30%. The dry matter (%) of plant was remarkably reduced with the severity of treatment and was associated with the appearance of necrotic leaves. These results indicated that salinity limited the biomass production of F. vulgare. In this context, a severe reduction in growth has been attributed to increases in Na+ and Cl- ions, accompanied by a major reduction in Ca2+and K+ ion concentrations in plant tissues (Valdez-Aguilar et al. 2009). Additionally, data in Table 1 indicated that the changes in salinity levels affected seed yield (g per Plant) of Foeniculum vulgare Mill. Thus the seed yield in general decreased under the various saline treatments. The ANOVA indicated that the decrease in seed yield was highly significant in salinity treatments. Concomitant with the decrease in seed yield, the number of umbels per plant, the 1000 seed weight as well as the number of branches of Turkish fennel,diminished significantly with the increasing of NaCl levels. In good agreement with our results, a decrease in yields and plant growth parameters of F. vulgare including plant height, fresh weight yield, biomass, 1000-fruit yield and seed yield, under salinity was reported by Semiz et al. (2012). The reduce of seed yield character under salt treatments may be due to exposure to injurious levels of salinity causing a decrease of turgor which would result in a decrease of growth and development of cells and might arise from a reduction of flower production and/or a decrease of their fertility.
According to Sreenivasulu et al. (2007) the adverse effects of salts on plant growth may be divided into three broad categories: (i) a reduction in osmotic potential of the soil solution that reduces plant available water and thus creates a water stress in plants, (ii) a deterioration in the physical structure of the soil such that water permeability and soil aeration are diminished and (iii) increase in the concentration of the certain ions that have an inhibitory effect on plant metabolism (specific ion toxicity) and mineral nutrient imbalances and deficiencies or (iv) a combination of these factors.
Table 1. Salinity effect on Foeniculum vulgarae Mill plant growth and yield components |
||||
|
0 mmol |
25 mmol |
50 mmol |
75 mmol |
Height (cm) |
68.06±0.33a |
60.15±0.41b |
55.34±0.66c |
47.09±±0.06d |
Fresh matter weight (g) |
28.04±0.10a |
26.15±0.05ab |
20.77±0.02b |
17.97±0.02c |
Dry matter weight (g) |
6.12±0.03a |
5.02±0.12b |
3.09±0.01c |
2.33±0.02d |
Dry matter % |
21.82±0.03a |
19.19±0.08b |
14.87±0.22c |
12.96±0.02c |
Seed yield per plant (g) |
9.33±0.02a |
8.46±0.04b |
6.03±0.08b |
5.84±0.05b |
Umbels/plant |
8.70±0.14a |
7.66±0.06b |
6.13±0.08c |
5.72±0.09d |
Number of branches |
10.54±0.33a |
9.05±0.02b |
8.11±0.01bc |
6.88±0.03c |
1000 seed weight (g) |
5.17±0.01a |
4.67± 0.01b |
4.19±0.03bc |
3.69± 0.01c |
Seed yield/plant (g) |
8.18± 0.03a |
7.22± 0.01a |
6.08±0.02 b |
5.79± 0.00b |
Values with different superscripts (a–d) are significantly different at p < 0.05 (means of six replicates ± S.D).
-
Salinity effect on oil yield and fatty acid composition
Lipids are the most effective sources of storage energy, they function as insulators of delicate internal organs and hormones, and they play important roles as the structural constituents of most of the cellular membranes (Singh et al. 2002). They also have vital roles in the tolerance to several physiological stressors in a variety of organisms.
Based on our experimental data, it was shown that the oil yield (%) of fennel seeds declines sharply with the severity of NaCl treatment. A progressive decrease with increasing salinity level was also observed. Thus, salt reduced oil yield on average 3.84%, 30.68% and 43.72%, respectively, with 25, 50 and 75 mM of NaCl, compared to control conditions (Table 2). In fennel seeds, the reduction of oil yield could be attributed to an inhibition of lipid biosynthesis and/or a stimulation of lipolytic and peroxidative activities under salt effect.
As it can be seen in Table 2, lipids extracted from fennel seeds are dominated by C18 fatty acids which is typical one in higher plants (Mazliak 1968). Analysis of fatty acid composition indicated that in controlled plants, petroselinic acid (C18:1 n12) was the major compound (75.43% of TFA) followed by linoleic (C18:2 n6) and oleic (C18:2 n9) acids, which constitute 8.04 and 7.56 % of TFA, respectively (Table 2). Fennel seeds were characterized by the presence of a high proportion of monounsaturated fatty acids (MUFAs) (91.03% of TFA). Polyunsaturated (PUFA) and saturated fatty acids (SFA) represented only 4.21 and 0.11 %, respectively.
It is well known that fatty acids, the main components of plasma membrane lipids, are considered important in salt tolerance of plants by maintaining the membrane fluidity (Malkit et al. 2002). As shown in Table 2, fatty acid composition of fennel seeds was modified by NaCl treatments. Salinity reduced the percentage of petroselinic (C18:1 n12) by 6.52; 19.77; 35.48 % and that of linoleic one by 2.11, 21.26 and 54.72 %, respectively, at 25, 50 and 75 mM NaCl. In addition, the level of oleic acid diminished especially in accordance to the degree of salt constraint. In contrast, the decrease of the level of petroselinic acid with raising NaCl concentrations was accompanied by a concomitant increase of palmitic acid proportion and the increases were 1.78; 2.66; 3.14 folds, respectively, at 25, 50 and 75 mM NaCl, in respect to the control. Therefore, environmental factors may affect fatty acids, not only by modifying enzyme activities but also by affecting fatty acid transport from the plastid. In developing seeds, fatty acid synthesis up to the formation of 18:1 occurs in plastids, while desaturation to 18:2 and 18:3 takes place in the cytosol (Flagella et al. 2004).
On other side, the degree of fatty acid unsaturation is important to maintain the membrane fluidity and to provide the appropriate environment for membrane functions. In addition, Unsaturation of fatty acids also counteracts salt stress. Hydrogen atoms adjacent to olefinic bonds are susceptible to oxidative attack. Lipids are rich in these bonds and are a primary target for oxidative reactions. In fennel seeds, under 25, 50 and 75mM, the unsaturated to saturated fatty acid (UFA/SFA) ratio was reduced from 21.64 to 2.18 which leads probably to a decrease in the passive membrane permeability. The effect of salinity on the degree of fatty acids unsaturation was assessed using the double bond index (DBI) calculated according to Rie De Vos et al. (1993). Results showed that this parameter decreased in comparison to the control. This result indicates that salinity affects the unsaturation degree of the fatty acids pool and thus the quality and the stability of the fennel oil. This fact could be explained by a possible reduction of the desaturase activity which appeared as an adaptative feature to salinity (Kuiper 1984). In fact, the ability to adjust membrane lipid fluidity by changing levels of unsaturated fatty acids is a feature of stress acclimating plants provided mainly by the regulated activity of fatty acid desaturases. In good agreement with our results, a decrease of unsaturation degree was observed in Coriandrum sativum(Naffati and Marzouk 2011), while it increased in Carthamus tinctorius(Harrathi et al. 2011) indicating that the response to salt constraint depends on plant species. In general, our results concerning the changes in fatty acids (especially the decrease of DBI and the reduction of the content of unsaturated fatty acids in seeds could represent an indirect evidence for the oxidative stress generated by salt stress.
Table 2. Effect of salinity on fatty acid composition and DBI changes from Foeniculum vulgarae Mill seeds. |
|||||||||||||||||||||||||||||||||||||||||||||||||||||||||||||||||||||||||||
|
|||||||||||||||||||||||||||||||||||||||||||||||||||||||||||||||||||||||||||
Values with different superscripts (a–d) are significantly different at p < 0.05 (means of six replicates). |
-
Salinity effect on essential oil yield and component proportions
As shown in Figure 1, in the control seeds, essential oil yield was 2.62%, based on their DW, and is significantly improved by the salt treatment. The application of increasing NaCl concentrations (25, 50, and 75 mM) gave essential oils with a yield of 2.84, 3.40, and 4.11%, respectively.
|
Fig. 3 Effect of salinity on essential oil yield (%) of Foeniculum vulgarae Mill seeds. Values with different superscripts (a–c) are significantly different at p < 0.05 (means of six replicates). |
It was well known that essential oil (EO) yield is influenced by intrinsic parameters (such as growth stages) and extrinsic ones (such as pedoclimatic conditions and salinity). Significant changes of EO yield (P< 0.05), as calculated on the basis of dry weight (DW) were observed among the different salt doses for EO yield (w/w). Similar results were recorded for fennel (Semiz et al. 2012) and coriander (Naffeti et al. 2011) seeds. The authors reported that under moderate NaCl concentration essential oil content increased. However, a regular decrease and the lowest oil content were obtained at the highest salinity concentration. The increase in oil content in some of the salt stressed plants might be attributed to decline the primary metabolites due to the effects of salinity, causing intermediary products to become available for secondary metabolites synthesis (Morales et al. 1993).
Changes in essential composition of fennel seeds under salt are illustrated in the Table 3. In the control seeds, 16 compounds were identified, accounting for 96.67% of total constituents. The essential oil was of the Trans-Anethol (84.20%) chemotype. Trans-Anethole is an isomer of methyl chavicol with only position of the double bound of the propenyl chain. Other main compounds were Fenchone(4.26%), Estragol(2.74%) and Limonene (2.01%). Trans-Anethol and Estragol are often the main fennel oil components. They are isomers with the only difference being the position of the double bound of the propenyl chain. The relative concentration of these compounds varies considerably depending on the phonological state and origin of the fennel. The essential oil composition of F. vulgare exhibits considerable chemodiversity depending upon the method of extraction and geographical origin. While Trans-Anethol is the main component in the studied essential oil, estragol constitutes the main component in the Turkish one (Telci et al. 2009).
Therefore, application of salinity induced marked changes in the essentialoil quality. The Estragol percentage increased withthe different treatments by about 2 folds at 25 mM NaCl and, 3.5 and 5.45 folds, at 50 and 75 mM NaCl, respectively. As well, salinity improved the percentage of Limoneneby about 3 folds, compared to the control at 75 mM NaCl. Also, under salt treatment, Fenchone percentage increases from 4.26% to 12.12 % at 75 mM NaCl, compared to the control. However, the salt stress decreased the level of Trans-Anethol under the different NaCl levels. Thus, salinity induced the modification of the essential oil compositon and this change will probably result in the modification of the essential oil odor. In fact, the effect of salinity on essential oil and its constituents may be due to its effects on enzyme activity and metabolism. Conversely, Abd El-Wahab (2006) reported a decrease in the essential oil yield of fennel and a reduction in Trans-Anethole percentage with saline water.
Thus, Tunisian F. vulgare seeds cultivated under NaCl salinity may provide a potential source of volatile substances. Major changes observed in the composition were due to the relative proportions of constituents and not to the presence of new or the absence of particular ones. As a whole, the stimulations in volatile oil composition, in F. vulgare seed tissues under salinity, support our assumption that plants tolerant to salt stress are potentially useful systems for the production of secondary metabolites to be used by both food and pharmaceutical industries.
Table 3. Proportion (%) changes of essential oil components in Foeniculum vulgarae Mill seeds as influenced by salinity.
|
||||||||||||||||||||||||||||||||||||||||||||||||||||||||||||||||||||||||||||||||||||||||||||||||||||||||||||||||||||||||||||||||||||||||||||||||
|
||||||||||||||||||||||||||||||||||||||||||||||||||||||||||||||||||||||||||||||||||||||||||||||||||||||||||||||||||||||||||||||||||||||||||||||||
MS, mass spectrum; Co-GC, co-injection with authentic compound. Values followed by the same letter in the columns in the rows did not share significant differences at 5% (Duncan test). (Means of six replicates ±S.D). Values with different letters (a–d) are significantly different at p < 0.05. a Retention indices calculated using an apolar column (HP-5) and polar column (HP-Innowax). b Retention indices calculated using an apolar column (HP-5) and polar column (HP-Innowax). c Retention indices relative to C8-C22 n-alkanes on the (HP-Innowax).
|
-
Conclusion
The present work has extended our knowledge on the effect of NaCl on biochemical composition of F. vulgare seeds, which provoked much interest as sources of natural products, mainly in food industry due to the presence of many useful compounds. From practical standpoint, our results revealed that NaCl treatments decreased the seeds yield of fennel, and altered the quality and the stability of the oil; in fact, the unsaturation degree of the fatty acid pool diminished. Moreover, salinity was found to significantly influence the secondary metabolites production. It stimulates the essential oil production. Therefore, the development of new chemotypes at different salt levels could be considered as valuable aspects of salinity stress in some plants inducing them to produce compounds with industrial and pharmaceutical interest. Finally, these biochemical changes induced by salinity could reflect an adaptation response.
-
References
Abd El-Wahab MA (2006) The efficiency of using saline and fresh water irrigation as alternating methods of irrigation on the productivity of Foeniculum vulgare Mill subsp. vulgare var. vulgare under North Sinai conditions. Res J Agr Biol Sci 2:571-7
Adams RP (2001) Identification of essential oil components by gas chromatography/quadrupole mass spectroscopy. Carol Stream IL, USA: Allured.
Arnon DI (1950) The Water Culture Method for Growing Plants without Soil. In: California Agricultural Experiment Station Circular 347. The College of Agriculture, University of California, Berkeley, CA, pp: 1-39.
Arzani A (2008) Improving salinity tolerance in crop plants: a biotechnological view. Vitro Cell Dev Biol Plant 44: 373-383.
Bâatour O, Mahmoudi H, Tarchoun I, Nasri N, Kaddour R, Zaghdoudi M, Wissal A, Hamdaoui G, Lachaâl M, Marzouk B (2012) Salt effect on phenolics and antioxidant activities of Tunisian and Canadian sweet marjoram (Origanum majorana L.) shoots. J Sci Food Agric 93: 134–141.
Bahmani KA, Izadi-Darbandi AA, Jafari SAS, Noori M, Farajpour M (2012)Assessment of genetic diversity in Iranian fennels using ISSR markers. J Agric Sci 4:79-84.
Bligh EG, Dyer WJ (1959) A rapid method for total lipid extraction and purification. Can J Biochem Physiol 37: 911-917
Bourgou S, Bettaieb I, Saidani M, Marzouk B (2010) Fatty acids, essential oil and phenolics modifications of black cumin fruit under NaCl stress conditions. J Agric Food Chem 58: 12399–12406.
Cecchi G, Biasini S, Castano J (1985) Méthanolyse rapide des huiles en solvant. Note de laboratoire. Rev Fr Corps Gras 4: 163–164.
Damjanovic B, Lepojevic Z, Zivkovic V, Tolic A (2005) Extraction of fennel (Foeniculum vulgare Mill.) seeds with supercritical CO2: Comparison with hydrodistillation. Food Chem 92:143-149.
El-Awadi ME, Hassan EA (2010)Physiological responses of fennel (Foeniculum vulgare Mill) plants to some growth substances. The effect of certain amino acids and a pyrimidine derivative. J Am Sci 6: 120-124.
Flagella Z, Giuliani MM, Rotunno T, Di Caterina R, De Caro A (2004) Effect of saline water on oil yield and quality of a high oleic sunflower (Helianthus annuus L.) hybrid. Eur J Agron 21: 267-272.
Harrathi J, Hosni K, Bouraoui KN, Attia H, Marzouk B, Magne C, Lachaal M (2011) Effect of salt stress on growth, fatty acids and essential oils in safflower (Carthamus tinctorius L.). Acta Physiol Plant 24: 129–137
Kuiper PJC (1984) Function of plant cell membranes under saline conditions: membrane lipid composition and ATPases. In: R. C. Staples, and G. H. Toenniesses (eds), Salinity Tolerance in Plants. 77-91.
Mahajan S, Tuteja N (2005). Cold, salinity and drought stresses: An overview. Arch Biochem Biophys 444:139-158
Malkit A, Sadka A, Fisher M, Goldshlag P, Gokhman I, Zamir A (2002) Salt induction of fatty acid elongase and membrane lipid modifications in the extreme halotolerant Alga Dunaliella salina. Plant Physiol 129: 1320–1329
Marzouk B, Cherif A (1981) La lipogenèse dans l’olive. I- Formation des lipides neutres. Oléagineux, 36: 77-82.
Mazliak P (1968) Le metabolisme des lipides dans les plantes superieures. Edition Masson and Cie, Paris.
Neffati M, Sriti J, Hamdaoui G, Kchouk ME, Marzouk B (2011) Salinity impact on fruit yield, essential oil composition and antioxidant activities of Coriandrum sativum fruit extracts. Food Chem 124: 221-225.
Piccaglia R, Marotti M (2001). Characterization of some Italian types of wild fennel (Foeniculum vulgare Mill.). J Agric Food Chem 49:239-244.
Rie De Vos CH, Ten Bookum WM, Voojis R, Schat H, Deko LJ (1993) Effect of copper on fatty acid composition and peroxydation of lipids in the roots of copper tolerant and sensitive Silene cucubalus. Plant Physiol Biochem 31: 151–158.
Semiz, GD, Ünlükara A, Yurtseven E, Suarez DL, Telci İ (2012) Salinity Impact on Yield, Water Use, Mineral and Essential Oil Content of Fennel (Foeniculum vulgare Mill.). Tarım Bilimleri Dergisi-J Agric Sci 18:177-186.
Singh, S.C., Sinha, R.P., Hader, D.P., (2002). Role of lipids and fatty acids in stress tolerance in cyanobacteria. Acta Protozool 41, 297–308.
Sreenivasulu N, Sopory SK, Kavi Kishor PB (2007) Deciphering the regulatory mechanisms of abiotic stress tolerance in plants by genomic approaches. Gene, 388: 1-13.
Telci I, Demirtas I, Sahin A (2009) Variation in plant properties and essential oil composition of sweet fennel (Foeniculum vulgare Mill.) fruits during stages of maturity. Ind Crops Prod 30: 126–130.
Valdez-Aguilar LA,Grieve CM, Poss J, Mellano M (2009).Hypersensitivity Hyper sensitivity of Ranunculus asiaticus to salinity and alkalinity in irrigation water in salt cultures. HortScience HortScience 44: 138-44.
Zhu JK (2007). Plant salt stress. In: Encyclopedia of Life Sciences. John Wiley and Sons, Ltd: Chichester www. Els. Net.