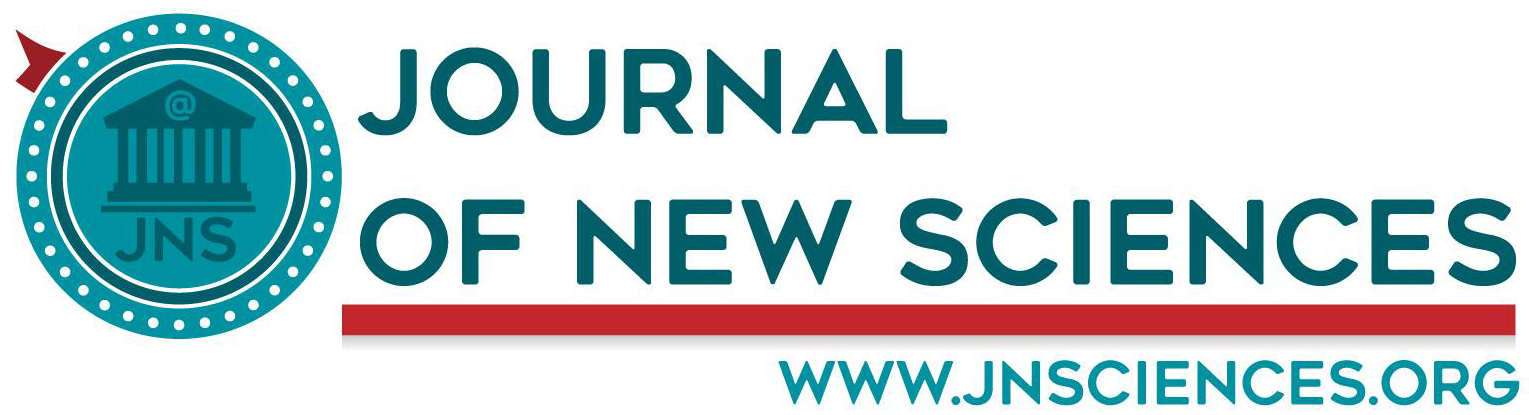
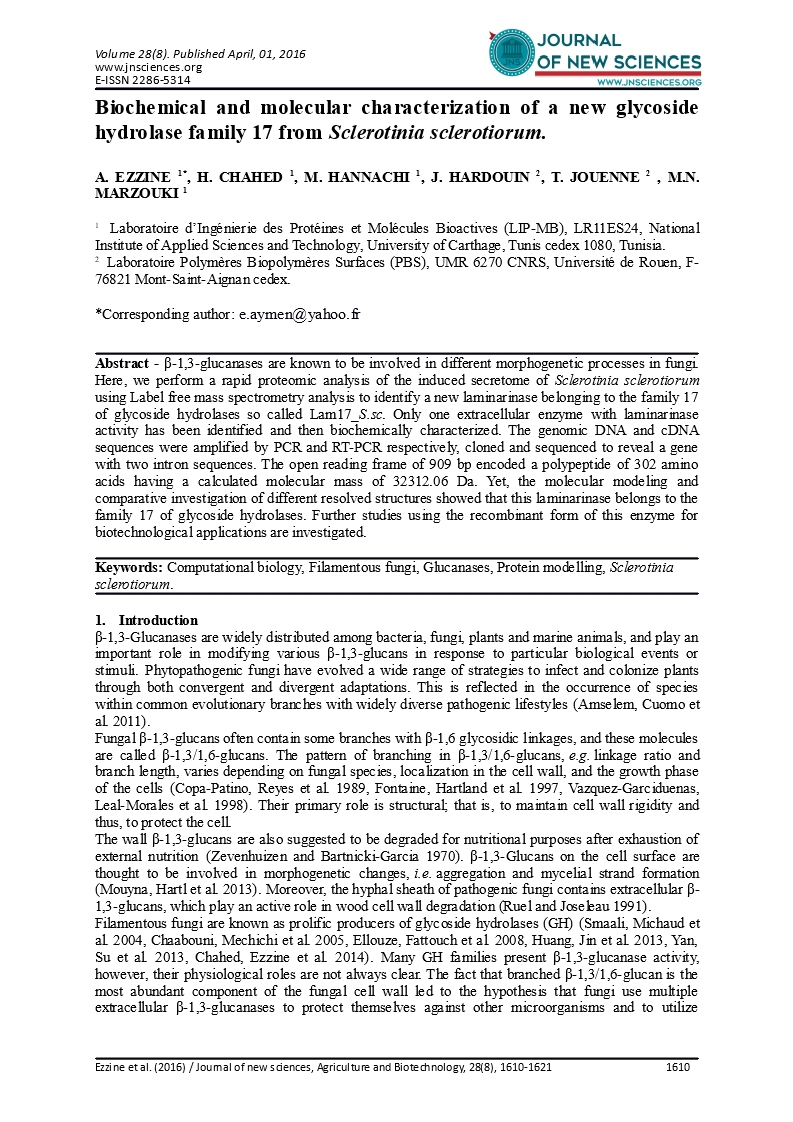
- Category: Volume 28
- Hits: 8000
Biochemical and molecular characterization of a new glycoside hydrolase family 17 from Sclerotinia sclerotiorum.
A. Ezzine 1*
H. Chahed 1
M. Hannachi 1
J. Hardouin 2
T. Jouenne 2
M.N. Marzouki 1
1 Laboratoire d’Ingénierie des Protéines et Molécules Bioactives (LIP-MB), LR11ES24, National Institute of Applied Sciences and Technology, University of Carthage, Tunis cedex 1080, Tunisia.
2 Laboratoire Polymères Biopolymères Surfaces (PBS), UMR 6270 CNRS, Université de Rouen, F-76821 Mont-Saint-Aignan cedex
Abstract - β-1,3-glucanases are known to be involved in different morphogenetic processes in fungi. Here, we perform a rapid proteomic analysis of the induced secretome of Sclerotinia sclerotiorum using Label free mass spectrometry analysis to identify a new laminarinase belonging to the family 17 of glycoside hydrolases so called Lam17_S.sc. Only one extracellular enzyme with laminarinase activity has been identified and then biochemically characterized. The genomic DNA and cDNA sequences were amplified by PCR and RT-PCR respectively, cloned and sequenced to reveal a gene with two intron sequences. The open reading frame of 909 bp encoded a polypeptide of 302 amino acids having a calculated molecular mass of 32312.06 Da. Yet, the molecular modeling and comparative investigation of different resolved structures showed that this laminarinase belongs to the family 17 of glycoside hydrolases. Further studies using the recombinant form of this enzyme for biotechnological applications are investigated.
Keywords: Computational biology, Filamentous fungi, Glucanases, Protein modelling, Sclerotinia sclerotiorum.
-
Introduction
β-1,3-Glucanases are widely distributed among bacteria, fungi, plants and marine animals, and play an important role in modifying various β-1,3-glucans in response to particular biological events or stimuli. Phytopathogenic fungi have evolved a wide range of strategies to infect and colonize plants through both convergent and divergent adaptations. This is reflected in the occurrence of species within common evolutionary branches with widely diverse pathogenic lifestyles (Amselem, Cuomo et al. 2011).
Fungal β-1,3-glucans often contain some branches with β-1,6 glycosidic linkages, and these molecules are called β-1,3/1,6-glucans. The pattern of branching in β-1,3/1,6-glucans, e.g. linkage ratio and branch length, varies depending on fungal species, localization in the cell wall, and the growth phase of the cells (Copa-Patino, Reyes et al. 1989, Fontaine, Hartland et al. 1997, Vazquez-Garciduenas, Leal-Morales et al. 1998). Their primary role is structural; that is, to maintain cell wall rigidity and thus, to protect the cell.
The wall β-1,3-glucans are also suggested to be degraded for nutritional purposes after exhaustion of external nutrition (Zevenhuizen and Bartnicki-Garcia 1970). β-1,3-Glucans on the cell surface are thought to be involved in morphogenetic changes, i.e. aggregation and mycelial strand formation (Mouyna, Hartl et al. 2013). Moreover, the hyphal sheath of pathogenic fungi contains extracellular β-1,3-glucans, which play an active role in wood cell wall degradation (Ruel and Joseleau 1991).
Filamentous fungi are known as prolific producers of glycoside hydrolases (GH) (Smaali, Michaud et al. 2004, Chaabouni, Mechichi et al. 2005, Ellouze, Fattouch et al. 2008, Huang, Jin et al. 2013, Yan, Su et al. 2013, Chahed, Ezzine et al. 2014). Many GH families present β-1,3-glucanase activity, however, their physiological roles are not always clear. The fact that branched β-1,3/1,6-glucan is the most abundant component of the fungal cell wall led to the hypothesis that fungi use multiple extracellular β-1,3-glucanases to protect themselves against other microorganisms and to utilize carbon sources during hyphal growth (Copa-Patino, Reyes et al. 1989, Fontaine, Hartland et al. 1997, Vazquez-Garciduenas, Leal-Morales et al. 1998). β-1,3-glucanases have been classified into endo- or exo-type enzymes depending on their mode of action, i.e., endo-type β-1,3-glucanase randomly hydrolyzes linear substrates, whereas exo-type enzymes releases glucose residues from the chain ends (Pitson, Seviour et al. 1993). In the carbohydrate-active enzymes (CAZy) data base (Henrissat and Bairoch 1993, Bourne and Henrissat 2001, Cantarel, Coutinho et al. 2009), enzymes having β-1,3 glucanase activity belong to the glycoside hydrolase families 5, 16, 17, 55, 64, 72, and 81.
β-1,3-Glucanases are often termed laminarinases since the most widely studied natural β-1,3-glucan-containing polymers is laminarin. Degradation of β-1,3-glucans by fungi often involves the cooperative action of multiple β-1,3-glucanases rather than a single enzyme. Although some fungal β-1,3-glucanases are constitutively produced, their expression levels are often controlled by culture conditions (Pitson, Seviour et al. 1993). Many β-1,3-glucanases have been characterized, and they exhibit a wide variety of substrate specificities and modes of actions (Martin, McDougall et al. 2007). These facts suggest the involvement of β-1,3-glucanases in mobilization of cell wall β-glucans especially in the case of yeast enzymes. However, in plants, glucanases are known by their protective role against fungal pathogens which was confirmed after their expression in a variety of plant species upon fungal invasion (Adams 2004).
Fungal β-1,3-Glucanases play important roles in morphogenetic processes, β-glucan mobilization, and fungal pathogen-plant interactions (Bachman and McClay 1996). When combined with chitinase, these enzymes demonstrated antimycotic activities that make them efficient biological control agents against fungal plant pathogens (Noronha and Ulhoa 1996, Zverlov, Volkov et al. 1997). Laminarinase was also used in algal biomass conversion to fermentable sugar (Sharma and Nakas 1987, Zverlov, Volkov et al. 1997). Furthermore, several applications were reported using this enzyme such as malting and brewing processes (Konig, Grasser et al. 2002), commercial yeast extract production (Ryan and Ward 1985), animal feed (Konig, Grasser et al. 2002), medical fields (Pang, Otaka et al. 2005).
Here, we explore the new developed technology label-free mass spectrometry to identify and characterize a new extracellular GH17 with laminarinase activity produced by Sclerotinia sclerotiorum.
-
Material and methods:
-
Chemical reagents and enzymes:
-
Laminarin, IPTG, X-Gal, DTT, ampicillin and Coomassie blue R-250 (Powder) were purchased from Sigma, Aldrich. Pfu DNA polymerase from BioBasic Inc., primers were from Invitrogen,
-
Organism and culture conditions:
S. sclerotiorum was maintained on potato dextrose agar medium (PDA). Actively growing fungal mycelium from a 3 days culture was transferred into 200 ml of enriched broth medium prepared according to Mandel's medium (Mandels and Weber 1969) supplemented with 1% (w/v) of laminarin before sterilization. After adding mycelium to the inducing medium, incubate on a rotary shaker at 150 rpm at 25°C for 5 days. The culture was centrifuged and the recovered supernatant was used as crude enzyme preparation.
-
Protein precipitation and laminarinase assay:
The produced extracellular proteins were precipitated using 80% ammonium sulfate and recovered by centrifugation at 7500 rpm for 45min at 4°C. The pellet was resuspended in 100mM sodium acetate buffer (pH 5.0).
The standard assay for β-1, 3-glucanase activity contained 100 µl of 1% laminarin, 20 µl enzyme solution (15 µg of protein), and 180 µl of 0.1M sodium acetate buffer (pH 5.0). The reaction was incubated at 37°C, for 10 min. Reducing sugars released from laminarin were measured by the method of Dygert et al. (1965) (Dygert, Li et al. 1965). One unit of β-1,3-glucanase was defined as the amount of enzyme releasing 1µmol of glucose/min under these conditions.
For optimal temperature determination, the activity assay was performed at incubation temperatures varying from 35 to 65°C with 5°C intervals during 30min.
The optimum pH was determined by incubating at 50°C in either 0.1M sodium citrate buffer (pH 3.5 to 5.0) or 0.1 M NaPO4 buffer (pH 6.0 to 8.0).
For metal ion effect measurement, the enzyme was pre-incubated for 60 min at room temperature with the following salts CaCl2, CuSO4, FeCl3, MgCl2, MnCl2, or ZnCl2 (1mM or 5mM) and the activity was assayed under the standard conditions using 1% laminarin as substrate.
-
Genomic and complementary DNA amplification and sequencing:
Genomic DNA of S. sclerotiorum was purified according to a modified protocol of Sambrook et al.(Sambrook, Fritsch et al. 1989) and used as template for a polymerase chain reaction amplification of the glucanase gene, using specific primers: Forward: 5’-ATGCGCTCGCTCGTTATTCTCCCG-3’ and Reverse: 5’-TTAACAATTCAACGAGTACTTGCTGC-3’. The amplification was performed with 2.5U of Pfu DNA polymerase to generate blunt ended PCR products.
The corresponding cDNA was synthesized by reverse transcription followed by PCR amplification (RT-PCR) using total RNA purified from laminarin-induced Sclerotinia culture. We used the same primers as in the amplification of genomic DNA. Amplified PCR products were purified from agarose gel using QIAquick Gel Extraction Kit (Qiagen) and inserted into SmaI site of the pUC19 vector (Invitrogen) then transformed into NEB5α competent cells (New England BioLabs). Several recombinant vectors obtained were sequenced from both ends using the universal M13 forward and reverse primers in an automated DNA sequencer (Model 370A, Applied Bio systems).
-
Screening for laminarinase isoforms by In-gel activity assay:
50µg of laminarin-induced crude extract were separated in 10% Native-PAGE. After migration, the polyacrylamide gel was cut into 2 mm fragments from its top to the bottom. Each fragment was ground in the activity buffer in order to elute the proteins from the gel. After a brief centrifugation, the soluble aliquotes were tested for β-glucanase activity with laminarin as substrate as described previously.
-
Mass spectrometry analysis:
-
Label-Free technique:
-
About 30 µg of proteins (from the crude extract) were resolved by 1D SDS-PAGE 7% and then briefly stained with Coomassie Blue. After destaining the gel, the protein band was excised and subjected to tryptic digestion. In brief, the disulfide bonds were reduced by the addition of dithiothreitol and the free cysteine residues were alkylated with iodoacetamide. The gel plugs were washed prior to a dehydration step, followed by the tryptic digestion. The resultant tryptic peptides were finally lyophilized and ready to use for the spectrometry analysis.
-
Database searches:
Raw data files were processed using Proteome Discoverer 1.3 software (Thermo Scientific). Peak lists were searched using the MASCOT search engine (Matrix Science) against the database NCBI (Taxonomy: other fungi). Database searches were performed with the following parameters: enzyme: trypsin; one missed cleavage site allowed; variable modifications: carbamidomethylation of cysteine and oxidation of methionine. The parent ion and daughter ion tolerances were 10 ppm and 0.5 Da, respectively. To avoid biased automatic annotation, peptide spectra for proteins of interest were manually inspected. To validate these peptides, reasonable coverage of b- and y-fragment ion series was required (at least 5 successive daughter ions).
-
Amino acid sequence analysis:
The corresponding amino acid sequence was deduced from the sequenced cDNA. Multalin software was used to generate the alignment of glucanases sequences (Corpet 1988). Rendering of the alignment figure including the prediction of the secondary structures was performed with the ESPript program (Gouet, Robert et al. 2003, Robert and Gouet 2014). Putative signal sequence was identified using the SignalP prediction software (Nielsen, Engelbrecht et al. 1997). The nucleotide sequence data of the GH17 of Sclerotinia sclerotiorum is available in the Gene Bank database (NCBI Reference Sequence: XM_001595467.1).
-
Model building of the tertiary structure of the glucanase:
The automated protein structure homology-modelling server, SWISS-MODEL (Schwede, Kopp et al. 2003) was used to generate the 3D model. The Deep View Swiss PDB Viewer software from EXPASY server (available at http://www.expasy.org/spdbv) was used to visualize and analyze the atomic structure of the model. Molecular modelling of S. sclerotiorum glucanase using SWISS-MODEL was analyzed based on the X-ray crystallographic structures of the GH17 of Banana (pdb accession code 2CYG). Finally, PyMOL presents the Molecular Graphic System used to render figures.
-
Results and discussion:
-
Label free mass spectrometry analysis and activity assay for the extracellular laminarinase produced by Sclerotinia sclerotiorum:
-
β-1,3-Glucan, a major component of the cell walls and resting structures of most fungi, is degraded by β-1,3-glucanases, which are grouped according to their mechanisms of hydrolysis 11. Endo-β-1,3-glucanases (EC 3.2.1.39) cleave the glucan chain at random sites. Exo-β-1,3-glucanases (EC 3.2.1.58) release glucose monomers from the non-reducing end of the glucan chain. Fungal non cellulolytic glucanases have been implicated as important factors in the mycoparasitic activities of various fungal species.
|
Figure 1: Extracellular laminarinase activity measured during S. sclerotiorum induced culture. Glucanase’s expression was induced by 1% laminarin (w/v) during 5 days of cultivation in enriched medium. The quantity of reducing sugars produced was determined according to Dinitrosalicylic acid assay method. One unit (U) of laminarinase activity was defined as the amount of enzyme releasing 1µmol of reducing sugar per min. Each value represents the average of three measurements. |
They have also a physiological role in morphologic and morpholytic processes during fungal development and differentiation and general cell wall modification. In Sclerotinia sclerotiorum, we can identify a complex system of extracellular enzymes with large spectral activities. Cellulolytic and non cellulolytic glucanases including β-1,4-; β-1,6- and β-1,3-Glucanases are the most identified.
When grown on laminarin as the sole carbon source, Sclerotinia sclerotiorum presents a laminarinase activity measured at its maximum after four days of induction (Figure 1). Compared to other β-1,3-glucanases, the extracellular activity measured here is relatively low. This could be attributed to the fact that Sclerotinia uses this enzyme activity in its own extracellular cell wall modification.
|
Figure 2: Screening for laminarinase isoforms into the secreted proteins. Secreted proteins from laminarin-induced Sclerotinia were separated with native-PAGE and then, the gel has been cut into fragments of 2mm. The activity of each eluted protein has been assayed and represented in function of the distance migrated. |
To identify and characterize the number of glucanase isoforms, if any, the S. sclerotiorum secreted proteins were analyzed by native PAGE. As shown in figure 2, the glucanase activities measured from cut bands revealed the presence of a unique laminarinase isoform under these induction conditions. This slab enzyme activity test is an efficient method to screen for different isoforms displayed in a protein mixture in a relatively fast way.
Furthermore, a Label-free mass spectrometry analysis was performed to the secreted protein mixture of S. sclerotiorum. This technique is used to identify few quantities of polypeptides into protein mixtures. Therefore, we applied this efficient technique to characterize all putative laminarinases induced and secreted by this pathogenic fungus (Figure S1). Results showed that only one putative Glucan β-1,3-glucosidase isoform that can exhibit laminarinase activity from secreted proteins. Two peptide sequences were identified by LC-MS/MS (Table 1).
Table 1: Sequence of internal peptides identified by Label-free mass spectrometry |
||
Experimental (Mw) |
Precursor (m/z) |
Peptide sequence |
P1: 1158,47124 |
579.235 |
- GYAASDCDTAK - |
P2: 1879,92075 |
626.64025 |
- GYAASDCDTAKQILPAAK - |
They belong to the amino acid sequence of the glucan β-1,3-glucosidase previously determined as a putative protein in the Gene Bank database. This polypeptide is formed by 302 amino acid residues and has a theoretical molecular weight of 32.3 kDa with a calculated pI of 4.78.
This analysis enabled us to confirm the presence of a single isoform of β-1,3-glucanase exhibiting laminarinase activity and consequently, we can directly use the crude extract without proceeding to purification steps, especially since the enzyme quantity and activity is also too low.
-
Optimal temperature and pH for the laminarinase activity:
The optimal S. sclerotiorum laminarinase activity was measured at pH 5 and 50°C (Figure 3A and 3B). Generally, fungal β-1,3glucanases present a maximum activity between pH 4 and 6 (Pitson, Seviour et al. 1993). When comparing the thermal stability of the S. sclerotiorum β-1,3-glucanase with those from other organisms, we can say that the S. sclerotiorum enzyme is relatively stable (Figure 3.C). It retained about 50% of its maximum activity when incubated at 50°C for 60 min. As to the β-1,3-glucanase of T. asperellum, it was stable for 90 min at 45°C and retained 57% of the maximum activity at 50°C (Bara, Lima et al. 2003).
Similarly, when the β-1,3-glucanase from A. fumigatus was pre-incubated for 1h at different temperatures, its stability was found to be markedly reduced at temperatures higher than the optimum temperature for activity (Fontaine, Hartland et al. 1997).
-
Metal ions effect on the extracellular laminarinase activity:
Using laminarin as assay substrate, a range of mono-, di and trivalent metal ions were examined for their effect on the extracellular β-1,3-glucanase activity from S. sclerotiorum.
Analysis of the results presented in Table 2 showed that the laminarinase activity was markedly sensitive to Mn2+, Cu2+ and Zn2+ divalent cations.
CuSO4 presented an important inhibitory effect on the extracellular S. sclerotiorum β-1,3-glucanase activity. However, MnCl2 increased significantly the laminarinase activity. These findings suggest the presence of an accessible, essential sulfhydryl or other readily oxidisable groups in this enzyme. The T. emersonii enzyme was also relatively unaffected by a range of metal ions and chemical modifiers (O'Connell, Piggott et al. 2011).
(A) (B)
(C) |
Figure 3: Influence of pH and temperature on the activity of extracellular Lam17_S.sc. (A) Enzyme activity was measured at different temperature from 35 to 65°C in 0.1M sodium acetate buffer (pH 5.0) for 5 min, using laminarin as substrate. (B) Optimum pH for the reaction determined by incubating the reaction at 50°C in either 0.1M sodium citrate buffer (pH 3.5 to 5.0) or 0.1 M NaPO4 buffer (pH 6.0 to 8.0). One unit of β-1,3-glucanase was defined as the amount of enzyme that released 1mmol of glucose/min under these conditions. (C) Thermal stability identification of the extracellular β-1,3-glucanase from S. sclerotiorum. The enzyme was pre-incubated at 40°C, 45°C, 50°C, 55°C or 60°C in 200 mM Tris-HCl buffer, pH 5.0. Residual activity was measured using laminarin as substrate. 100% represents the maximum activity measured in optimal conditions of temperature and pH. |
Table 2: Effect of selected metal ions on the laminarinase activity of the extracellular β-1, 3-glucanase isolated from S. sclerotiorum. |
||||
Reagents |
Relative activity % (a) (Concentration 1mM) |
Confidence interval |
Relative activity % (a) (Concentration 5mM) |
Confidence interval |
CaCl2 |
103.4 |
±3.09 |
102.1 |
±0.50 |
MgCl2 |
103.7 |
±4.04 |
104.0 |
±1.19 |
MnCl2 |
119 |
±3.55 |
144.7 |
±2.04 |
ZnSO4 |
104 |
±1.76 |
86.7 |
±0.80 |
CuSO4 |
81.9 |
±1.78 |
37.3 |
±0.24 |
FeCl3 |
115.4 |
±1.22 |
94.1 |
±0.83 |
Control |
100.0 |
±0.04 |
100.0 |
±0.04 |
(a): Results are the mean values of three replicates |
-
Molecular characterization and amino acid sequence analysis of the β-1,3-glucanase isoform:
Genomic DNA and cDNA of the S. sclerotiorum β-1,3 glucanase were amplified using the corresponding genome and total RNA, respectively. According to figure 4-A, we succeeded to amplify fragments of 1090 bp and 909 bp corresponding to the expected sizes of the genomic DNA and cDNA of the Glucan β-1,3 glucosidase sequences, respectively. Nucleotide sequences of the obtained PCR products were subsequently cloned and sequenced.
|
||||
|
||||
Figure 4: Amplification of nucleotide sequences of the Lam17_S.sc. (A): Line 1: 1Kb DNA ladder marker, line 2: RT-PCR amplification of the glucanase coding sequence was performed in two steps: reverse transcription of the total RNA collected from laminarin induced mycelia then the synthesized cDNA was used as template in PCR reaction for specific amplification of the coding sequence of interest. Line 3: The genomic DNA has been amplified by direct PCR using the genomic DNA of S. sclerotiorum. (B): Nucleotide sequence of the genomic DNA with introns mentioned with gray background. |
The resulted sequences were aligned and two introns were identified (figure 4-B). This result confirmed the theoretical gene sequence reported after the S. sclerotiorum whole genome sequencing. This sequence is available under the NCBI Reference: XM_001595467.1. Translation of the open reading frame provides the same glucanase amino acid sequence referenced in the database. Hence, the predicted protein sequence is composed of 302 amino acids and has a calculated molecular weight of 32311.9 Da and an isoelectric pH of 4.57.
|
Figure 5: Protein sequence analysis and secondary structure prediction of the Lam17_S.sc enzyme from S. sclerotiorum using ESPript program. Residues identical in all sequences are printed in white on a red background. Residues identical or with a conservative substitution in at least two thirds of the sequences are printed in red on a white background. A low consensus value (50%) was used in the multiple sequence alignment. A residue that is highly conserved appears as an uppercase letter in the consensus line. A residue that is weakly conserved appears as a lower-case letter in the consensus line. A position with no conserved residue is represented by a dot in the consensus line. Clusters of homologous symbols are indicated in the consensus line: IV (!), BDENZQ (#), LM ($) and FY (%). 3D structure is indicated at the top of the alignment. α-Helices, η-helices, β-sheets and strict β-turns are denoted α, η, β and TT, respectively. Signal peptide is in box. Sclerotinia sclerotiorum exhibited an identity of 90%, 75%, 69%, 65%, 64% and 64% with Hypothetical protein BC1G_08370 from Botrytis cinerea (gi|154304200), (Trans)glycosidase from Glarea lozoyensis ATCC 20868 (gi|512208181), Glycosyl hydrolase family 17 of Pseudogymnoascus destructans 20631-21 (gi|440636007), Glucan-β-glucosidase from Ophiostoma piceae UAMH 11346 (gi|512188771), Glycoside hydrolase family 17 protein from Baudoinia compniacensis UAMH 10762 (gi|627797125) and Glycosyl hydrolase family 17 from Colletotrichum higginsianum (gi|380492167), respectively. |
These data are in perfect correlation with the label free mass spectrometry data and confirm that we have characterized the only laminarinase isoform secreted under these conditions of induction.
Multiple alignment of the amino acid sequence of the studied glucanase with proteins database show that this enzyme belongs to the super-family 17 of glycosyl hydrolases (GH).
The ESPript analysis program allowed the prediction of the laminarinase secondary structure based on the multiple alignments to proteins with defined structures (Figure 5).
Sequence data analysis defined the studied laminarinase as an exo β-1, 3 glucanase having 75% homology with the one from Glarea lozoyensis 74030 (gb: EHK99043.1) and also 75% similarity with the transglycosidase from Glarea lozoyensis ATCC 20868 (gb: XP_008076313.1).
This prediction study elucidates the presence of eight β-sheets and eight α-helices localized in conserved regions. This typical (β/α)8 TIM-barrel motif found in GH17 consists of an internal crown of eight β-strands connected by more or less extended loops to an outer crown of eight α-helices. An additional C-terminal loop containing generally a β-sheet formed by two short antiparallel strands protrudes from the external crown of α-helices.
Hence, we can confirm that our laminarinase enzyme belongs to the family 17 of glycoside hydrolases and for this reason we can name it as Lam17_S.sc.
-
Modeling structure of the laminarinase enzyme:
A modeling study has been performed with RaptorX program to predict the 3D structure of the studied laminarinase. The model is shown to have a typical structure similar to GH17 enzymes based on the resolved crystal structure of the one from banana (2CYG) performed at 1.45Å resolution (Receveur-Brechot, Czjzek et al. 2006).
|
Figure 6: Model structure of the GH17 from Sclerotinia sclerotiorum. The structure is shown as cartoon. Chains are colored in chainbows. The overall structure is represented by a TIM-barrel (β/α)8 motif with long interconnecting loops. |
This program permitted to edit a predicted structure that matches with the typical GH17 tertiary structure presented by a TIM-barrel with a (β/α)8 motif (Figure 6). SignalP prediction analysis showed that the N-terminus of Lam17_S.sc contains a signal sequence for extracellular expression (figure 7-A). In the maturation steps and secretion procedures, the signal peptide is automatically removed to release the active protein (Figure 7-B). Based on the RaptorX program criterions, the created 3D model has a high indicator for a good quality. The predicted binding residues, colored in green into the surface defined model, are potentially involved in different substrates binding and stacking of the sugar rings of the substrate (S119, W155, F179, W182, F263, K271, P272, E284, and W287). Moreover, K29, Y60, E268 and K271 are also suggested to be putative catalytic residues for different ligands like β-D-Glucose.
|
Figure 7: Surface presentation of Lam17_S.sc. (A): Structure of the main protein shows the glucanase domain (in magenta) and the signal peptide colored in bleu. This signal peptide is used in the extracellular secretion pathway and is removed during maturation steps. (B): A groove exists along the surface of the enzyme and contains the catalytic residues E120 and E222 (in red). Some amino acids predicted as binding residues for stacking sugars and carbohydrates are mentioned with different colors. The aromatic residues Y60, W155, F179, W182, F263 and W287 are drawn in green color and other residues (K29, S119, K271, P272, E284 E268 and K271) are drawn in magenta in the surface presentation. |
In the catalytic groove there are two glutamate residues (E120, E222) which represent potentially the catalytic nucleophiles of Lam17_S.sc based on the primary and tertiary structures similarities with GH17 enzymes and Clan A of β-glycosidases. In general, the 5.5 Å distance between the two catalytic glutamate residues is typical of retaining enzymes (Henrissat, Callebaut et al. 1995). The distance for the putatively available glutamates was 5.4 Å between Glu120 and Glu222 (data not shown). Hence, they probably form the catalytic residues for retaining mechanism as known for β-1, 3 glucan hydrolysis processes.
-
Conclusion:
β-1,3-Glucans on the cell surface of fungi are thought to be involved in morphogenetic changes, i.e. aggregation and mycelial strand formation. Moreover, the hyphal sheath of pathogenic fungi contains extracellular β-1,3-glucanases, which play an active role in wood cell wall degradation (Ruel and Joseleau 1991). In this paper we studied the whole extracellular laminarinase activity produced by only one Glucan β-1,3-glucosidase enzyme secreted by laminarin induced S. sclerotiorum and named Lam17_S.sc. In-gel activity method and label free mass spectrometry techniques gave us a real fast way to confirm that there is only one β-1,3-glucanase isoform secreted into the supernatant of induced Sclerotinia. Modelling assay and structure prediction lead to define our enzyme as a GH17-like enzyme with a laminarinase activity. The catalytic groove and residues were predicted for a retaining mechanism of hydrolysis. The fact that typical structures and critical amino acid residues are conserved in Lam17_S.sc supports the likelihood that this enzyme can exhibit a potential exo-β-1,3-glucanase and β-1,3-glucanosyltransglycosylase.
The family of GH17 is determined to be involved in the defense of plants against pathogens through its ability to degrade fungal cell wall polysaccharides. In yeast this enzyme plays a role in cell expansion during growth, in cell-cell fusion during mating, and in spore release during sporulation. However, in fungi, the role of such enzymes still not very well studied. It is predicted to be the same role as in yeast cells. In the light of these suggestions, improved experiences will be investigated to more characterize this GH17 enzyme essentially under recombinant form.
Acknowledgment:
This work is entirely supported by the Laboratory of Protein Engineering and Bioactive Molecules (LIP-MB) in the National Institute of Applied Sciences and Technology of Tunis, University of Carthage, Tunisia.
-
References:
Adams, D. J. (2004). "Fungal cell wall chitinases and glucanases." Microbiology 150(Pt 7): 2029-2035.
Amselem, J., C. A. Cuomo, J. A. van Kan, M. Viaud, E. P. Benito, A. Couloux, P. M. Coutinho, R. P. de Vries, P. S. Dyer, S. Fillinger, E. Fournier, L. Gout, M. Hahn, L. Kohn, N. Lapalu, K. M. Plummer, J. M. Pradier, E. Quevillon, A. Sharon, A. Simon, A. ten Have, B. Tudzynski, P. Tudzynski, P. Wincker, M. Andrew, V. Anthouard, R. E. Beever, R. Beffa, I. Benoit, O. Bouzid, B. Brault, Z. Chen, M. Choquer, J. Collemare, P. Cotton, E. G. Danchin, C. Da Silva, A. Gautier, C. Giraud, T. Giraud, C. Gonzalez, S. Grossetete, U. Guldener, B. Henrissat, B. J. Howlett, C. Kodira, M. Kretschmer, A. Lappartient, M. Leroch, C. Levis, E. Mauceli, C. Neuveglise, B. Oeser, M. Pearson, J. Poulain, N. Poussereau, H. Quesneville, C. Rascle, J. Schumacher, B. Segurens, A. Sexton, E. Silva, C. Sirven, D. M. Soanes, N. J. Talbot, M. Templeton, C. Yandava, O. Yarden, Q. Zeng, J. A. Rollins, M. H. Lebrun and M. Dickman (2011). "Genomic analysis of the necrotrophic fungal pathogens Sclerotinia sclerotiorum and Botrytis cinerea." PLoS Genet 7(8): e1002230.
Bachman, E. S. and D. R. McClay (1996). "Molecular cloning of the first metazoan beta-1,3 glucanase from eggs of the sea urchin Strongylocentrotus purpuratus." Proc Natl Acad Sci U S A 93(13): 6808-6813.
Bara, M. T., A. L. Lima and C. J. Ulhoa (2003). "Purification and characterization of an exo-beta-1,3-glucanase produced by Trichoderma asperellum." FEMS Microbiol Lett 219(1): 81-85.
Bourne, Y. and B. Henrissat (2001). "Glycoside hydrolases and glycosyltransferases: families and functional modules." Curr Opin Struct Biol 11(5): 593-600.
Cantarel, B. L., P. M. Coutinho, C. Rancurel, T. Bernard, V. Lombard and B. Henrissat (2009). "The Carbohydrate-Active EnZymes database (CAZy): an expert resource for Glycogenomics." Nucleic Acids Res 37(Database issue): D233-238.
Chaabouni, S. E., T. Mechichi, F. Limam and N. Marzouki (2005). "Purification and characterization of two low molecular weight endoglucanases produced by Penicillium occitanis mutant Pol 6." Appl Biochem Biotechnol 125(2): 99-112.
Chahed, H., A. Ezzine, A. B. Mlouka, J. Hardouin, T. Jouenne and M. N. Marzouki (2014). "Biochemical characterization, molecular cloning, and structural modeling of an interesting beta-1,4-glucanase from Sclerotinia sclerotiorum." Mol Biotechnol 56(4): 340-350.
Copa-Patino, J. L., F. Reyes and M. I. Perez-Leblic (1989). "Purification and properties of a 1,3-beta-glucanase from Penicillium oxalicum autolysates." FEMS Microbiol Lett 53(3): 285-291.
Corpet, F. (1988). "Multiple sequence alignment with hierarchical clustering." Nucleic Acids Res 16(22): 10881-10890.
Dygert, S., L. H. Li, D. Florida and J. A. Thoma (1965). "Determination of reducing sugar with improved precision." Anal Biochem 13(3): 367-374.
Ellouze, O., S. Fattouch, F. Mestiri, M. R. Aniba and M. N. Marzouki (2008). "Optimization of extracellular xylanase production by Sclerotinia sclerotiorum S2 using factorial design." Indian J Biochem Biophys 45(6): 404-409.
Fontaine, T., R. P. Hartland, A. Beauvais, M. Diaquin and J. P. Latge (1997). "Purification and characterization of an endo-1,3-beta-glucanase from Aspergillus fumigatus." Eur J Biochem 243(1-2): 315-321.
Fontaine, T., R. P. Hartland, M. Diaquin, C. Simenel and J. P. Latge (1997). "Differential patterns of activity displayed by two exo-beta-1,3-glucanases associated with the Aspergillus fumigatus cell wall." J Bacteriol 179(10): 3154-3163.
Gouet, P., X. Robert and E. Courcelle (2003). "ESPript/ENDscript: Extracting and rendering sequence and 3D information from atomic structures of proteins." Nucleic Acids Res 31(13): 3320-3323.
Henrissat, B. and A. Bairoch (1993). "New families in the classification of glycosyl hydrolases based on amino acid sequence similarities." Biochem J 293 ( Pt 3): 781-788.
Henrissat, B., I. Callebaut, S. Fabrega, P. Lehn, J. P. Mornon and G. Davies (1995). "Conserved catalytic machinery and the prediction of a common fold for several families of glycosyl hydrolases." Proc Natl Acad Sci U S A 92(15): 7090-7094.
Huang, Y., Y. Jin, W. Shen, Y. Fang, G. Zhang and H. Zhao (2013). "The use of plant cell wall-degrading enzymes from newly isolated Penicillium ochrochloron Biourge for viscosity reduction in ethanol production with fresh sweet potato tubers as feedstock." Biotechnol Appl Biochem.
Konig, J., R. Grasser, H. Pikor and K. Vogel (2002). "Determination of xylanase, beta-glucanase, and cellulase activity." Anal Bioanal Chem 374(1): 80-87.
Mandels, M. and J. Weber (1969). "The production of cellulases." Adv. Chem. Ser. 95: 391-414.
Martin, K., B. M. McDougall, S. McIlroy, J. Chen and R. J. Seviour (2007). "Biochemistry and molecular biology of exocellular fungal beta-(1,3)- and beta-(1,6)-glucanases." FEMS Microbiol Rev 31(2): 168-192.
Mouyna, I., L. Hartl and J. P. Latge (2013). "beta-1,3-glucan modifying enzymes in Aspergillus fumigatus." Front Microbiol 4: 81.
Nielsen, H., J. Engelbrecht, S. Brunak and G. von Heijne (1997). "A neural network method for identification of prokaryotic and eukaryotic signal peptides and prediction of their cleavage sites." Int J Neural Syst 8(5-6): 581-599.
Noronha, E. F. and C. J. Ulhoa (1996). "Purification and characterization of an endo-β-1,3-glucanase from Trichoderma harzianum." Can. J. Microbiol 42: 1039-1044.
O'Connell, E., C. Piggott and M. Tuohy (2011). "Purification of exo-1,3-beta-glucanase, a new extracellular glucanolytic enzyme from Talaromyces emersonii." Appl Microbiol Biotechnol 89(3): 685-696.
Pang, Z., K. Otaka, T. Maoka, K. Hidaka, S. Ishijima, M. Oda and M. Ohnishi (2005). "Structure of beta-glucan oligomer from laminarin and its effect on human monocytes to inhibit the proliferation of U937 cells." Biosci Biotechnol Biochem 69(3): 553-558.
Pitson, S. M., R. J. Seviour and B. M. McDougall (1993). "Noncellulolytic fungal beta-glucanases: their physiology and regulation." Enzyme Microb Technol 15(3): 178-192.
Receveur-Brechot, V., M. Czjzek, A. Barre, A. Roussel, W. J. Peumans, E. J. Van Damme and P. Rouge (2006). "Crystal structure at 1.45-A resolution of the major allergen endo-beta-1,3-glucanase of banana as a molecular basis for the latex-fruit syndrome." Proteins 63(1): 235-242.
Robert, X. and P. Gouet (2014). "Deciphering key features in protein structures with the new ENDscript server." Nucleic Acids Res 42(Web Server issue): W320-324.
Ruel, K. and J. P. Joseleau (1991). "Involvement of an Extracellular Glucan Sheath during Degradation of Populus Wood by Phanerochaete chrysosporium." Appl Environ Microbiol 57(2): 374-384.
Ryan, E. M. and O. P. Ward (1985). "Study of the effect of β-1,3-glucanase fromBasidiomycete QM 806 on yeast extract production." Biotechnology Letters 7(6): 409-412.
Sambrook, J., E. F. Fritsch and T. Maniatis (1989). "Molecular cloning: a laboratory manual." Cold Spring Harbor Laboratory Press, NY 2nd ed.
Schwede, T., J. Kopp, N. Guex and M. C. Peitsch (2003). "SWISS-MODEL: An automated protein homology-modeling server." Nucleic Acids Res 31(13): 3381-3385.
Sharma, A. and J. P. Nakas (1987). "Preliminary characterization of laminarinase from Trichoderma longibrachiatum." Enzyme Microb Technol 9: 89-93.
Smaali, M. I., N. Michaud, N. Marzouki, M. D. Legoy and T. Maugard (2004). "Comparison of two beta-glucosidases for the enzymatic synthesis of beta-(1-6)-beta-(1-3)-gluco-oligosaccharides." Biotechnol Lett 26(8): 675-679.
Vazquez-Garciduenas, S., C. A. Leal-Morales and A. Herrera-Estrella (1998). "Analysis of the beta-1,3-Glucanolytic System of the Biocontrol Agent Trichoderma harzianum." Appl Environ Microbiol 64(4): 1442-1446.
Yan, P., L. Su, J. Chen and J. Wu (2013). "Heterologous expression and biochemical characterization of an endo-beta-1,4-glucanase from Thermobifida fusca." Biotechnol Appl Biochem 60(3): 348-355.
Zevenhuizen, L. P. and S. Bartnicki-Garcia (1970). "Structure and role of a soluble cytoplasmic glucan from Phytophthora cinnamomi." J Gen Microbiol 61(2): 183-188.
Zverlov, V. V., I. Y. Volkov, T. V. Velikodvorskaya and W. H. Schwarz (1997). "Highly thermostable endo-1,3-beta-glucanase (laminarinase) LamA from Thermotoga neapolitana: nucleotide sequence of the gene and characterization of the recombinant gene product." Microbiology 143 ( Pt 5): 1701-1708.