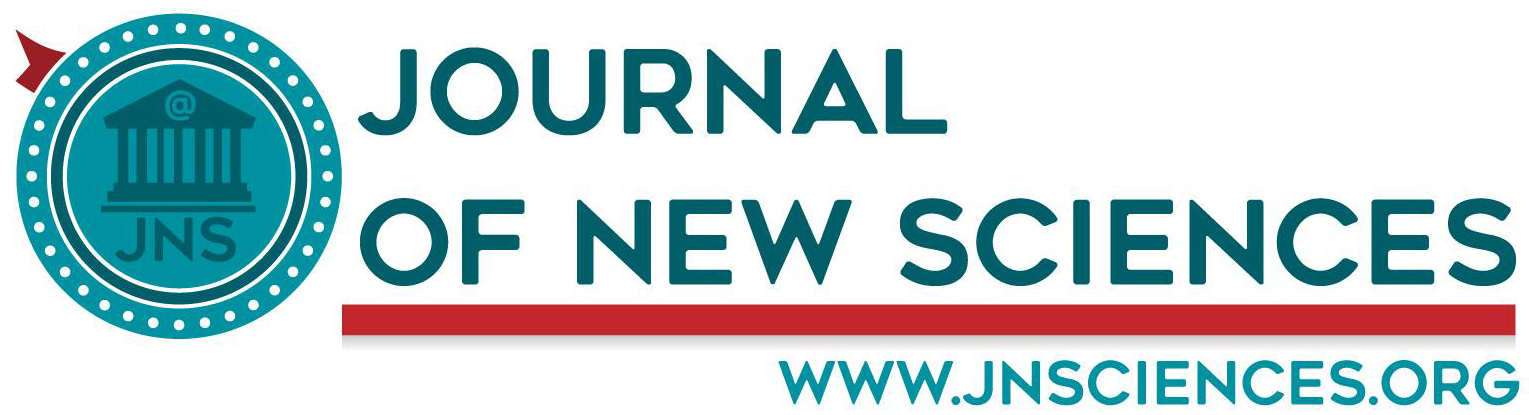
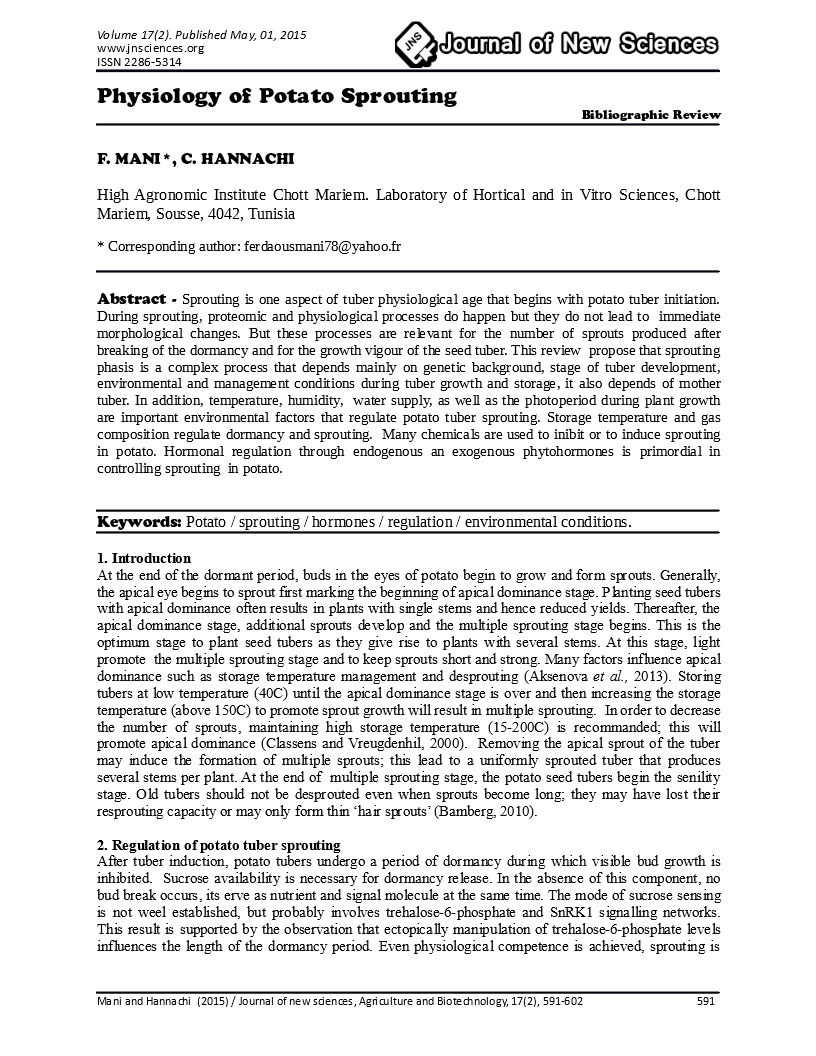
- Category: Volume 17
- Hits: 36230
Physiology of Potato Sprouting
Bibliographic Review
F. Mani*
C. Hannachi
High Agronomic Institute Chott Mariem. Laboratory of Hortical and in Vitro Sciences, Chott Mariem, Sousse, 4042, Tunisia
Abstract - Sprouting is one aspect of tuber physiological age that begins with potato tuber initiation. During sprouting, proteomic and physiological processes do happen but they do not lead to immediate morphological changes. But these processes are relevant for the number of sprouts produced after breaking of the dormancy and for the growth vigour of the seed tuber. This review propose that sprouting phasis is a complex process that depends mainly on genetic background, stage of tuber development, environmental and management conditions during tuber growth and storage, it also depends of mother tuber. In addition, temperature, humidity, water supply, as well as the photoperiod during plant growth are important environmental factors that regulate potato tuber sprouting. Storage temperature and gas composition regulate dormancy and sprouting. Many chemicals are used to inibit or to induce sprouting in potato. Hormonal regulation through endogenous an exogenous phytohormones is primordial in controlling sprouting in potato.
Keywords: Potato / sprouting / hormones / regulation / environmental conditions.
1. Introduction
At the end of the dormant period, buds in the eyes of potato begin to grow and form sprouts. Generally, the apical eye begins to sprout first marking the beginning of apical dominance stage. Planting seed tubers with apical dominance often results in plants with single stems and hence reduced yields. Thereafter, the apical dominance stage, additional sprouts develop and the multiple sprouting stage begins. This is the optimum stage to plant seed tubers as they give rise to plants with several stems. At this stage, light promote the multiple sprouting stage and to keep sprouts short and strong. Many factors influence apical dominance such as storage temperature management and desprouting (Aksenova et al., 2013). Storing tubers at low temperature (40C) until the apical dominance stage is over and then increasing the storage temperature (above 150C) to promote sprout growth will result in multiple sprouting. In order to decrease the number of sprouts, maintaining high storage temperature (15-200C) is recommanded; this will promote apical dominance (Classens and Vreugdenhil, 2000). Removing the apical sprout of the tuber may induce the formation of multiple sprouts; this lead to a uniformly sprouted tuber that produces several stems per plant. At the end of multiple sprouting stage, the potato seed tubers begin the senility stage. Old tubers should not be desprouted even when sprouts become long; they may have lost their resprouting capacity or may only form thin ‘hair sprouts’ (Bamberg, 2010).
2. Regulation of potato tuber sprouting
After tuber induction, potato tubers undergo a period of dormancy during which visible bud growth is inhibited. Sucrose availability is necessary for dormancy release. In the absence of this component, no bud break occurs, its erve as nutrient and signal molecule at the same time. The mode of sucrose sensing is not weel established, but probably involves trehalose-6-phosphate and SnRK1 signalling networks. This result is supported by the observation that ectopically manipulation of trehalose-6-phosphate levels influences the length of the dormancy period. Even physiological competence is achieved, sprouting is controlled by the level of phytohormones. Two phytohormones, ABA and ethylene, are supposed to suppress tuber sprouting; howreve, the exact role of ethylene remains to be investigated. Both Cytokinins and gibberellins are required for bud break and sprout growth, respectively. The fifth classical phytohormone, auxin, seems to play a role in vascular development. During the dormancy period, buds are symplastically isolated, which changes during bud break. Furthermore, vascular tissue develops below the growing bud most likely to support the outgrowing sprout with assimilates mobilised in parenchyma cells. So sprouting leads to major quality losses of stored potato tubers. However, control of tuber sprouting is a major objective in potato breeding. Even comparative transcriptome analysis revealed a large number of genes differentially expressed in growing versus dormant buds, no master-regulator of potato tuber sprouting has been identified so far (Sonnewald and Sonnewald, 2014). Application of gibberellic acid at low concentrations (5 and 10 mg/lit) promote sprouting and productivity of seed tubers of potatoes. Seed tuber production was increased by application of using gibberellic acid in all cultivars (Biemelt et al., 2004 ; Chapman, 2006). It also decreased, starch content and increased total content of sugar in potato tuber. Moreover, tubers treated with GA3 sprouted earlier while in control sprouting was very late and slow. We point that sugar content is one of the important parameters determining the sprouting of seed potatoes (Vreugdenhil and Struik, 2006 ; Barani et al., 2013). Previously it has been demonstrated that dormancy of whole potato tuber was totally broken with benzyladenine (BA) at a concentration of 20 ppm used for 24 h (Suttle, 2004). In This studies, the authors concluded that application of cytokinins resulted in the termination of dormancy and enhanced sprouting of potato tuber. Later, other physiological studies support the involvement of polyamines in several plant processes (Tiburcio et al., 2002) including tuber development (Roy and Nu, 2001 ; Mehta et al., 2002). In fact, Benzyladenine and spermine used alone significantly enhanced sprouting and germination in both potato cultivars, while cycocel inhibited sprouting tubers. Besides, the intensity of sprouting was less in combined treatments of Benzyladenine, spermine and cycocel. The combined treatments of Benzyladenine, spermine and cycocel showed an important increase in potato stolon and tuber formation. Although all these treatments increased chlorophyll contents, Benzyladenine alone overcome other treatments. Nodal parts of tubers showed comparatively greater IAA and GA contents than that of parts without eyes (Abid and Asghari, 2006). During sprouting, the tropinone reductase (EC 1.1.1.206) was detected in protein extracts of tuber tissues, but mostly in levels too low to be localised in individual cells. The function of this enzyme in potato that does not form hyoscyamine is not clear. The pseudotropine-forming tropinone reductase (EC 1.1.1.236) was detected in potato roots, stolons, and tuber sprouts. Cortex cells of root and stolon contained the protein; additional strong immuno-labelling was located in phloem parenchyma. In tuber spouts, however, the protein was detected in companion cells (Kaiser et al., 2006).
3. Effect of pre- and post-harvest environment on potato sprouting
At harvest, potato (Solanum tuberosum L.) tubers will not sprout even if placed in suitable environmental conditions for growth (Delaplace et al., 2008). This state can be affected by both the pre- and post-harvest environment (Suttle, 2004) and differs among cultivars (Hay and Porter, 2006 ; Carli et al., 2010). Otherwise, for seed potato tubers that are planted soon after their harvest it is necessary to have them primed to sprout. In some varieties sprouting can be hastened by storage at high temperatures. Therefore, sprouting is quicker after cold or heat shock during the early storage period. Struik (2006), found „Desirée‟ potatoes sprouted at 4 ,64 °Cd after two weeks of storage at 4°C followed by three weeks of warm-up at 20°C. This compares with 1,168 °Cd when initial storage was for two weeks at 20°Cfollowed by another three weeks at 4°C. The onset of sprout growth is also determinated ed by the development stage (physiological age) of the potato tuber (Struik and Wiersema, 1999). The evolution from physiologically young to physiologically old tubers affects sprout of tubers. These include date of emergence, stem numbers, canopy growth pattern, maturity date, total tuber yield and tuber size distribution (Christiansen et al., 2006). The same authors showed that the initiation of flower primordia may commence on sprouts before planting. This means that in some cultivars, and under certain storage conditions, the size of the first level of the main stem of the following potato plants is determined before planting. The determination of sprout behaviour during storage contributes to understanding the physiological ageing process of seed potato tubers and can be used to develop appropriate storage strategies prior to planting (Oliveira et al., 2012). Similary, potato varieties that have reached the end of “normal” sprouting, are recommended for early maturing crops, because tuber initiation starts earlier and the crop matures earlier. But, potato varieties that are at the end of their apical dominance period are recommended for medium and late maturing crops, since they mature later (Carli et al., 2010). This suggests that the duration from sprout initiation to planting time was an important determinant of the number of nodes on main shoot stems (Hay and Porter, 2006). Pre-sprouting methods are important in areas with a short growing season and in organic farming. Pre-sprouted seed tubers have earlier and faster tuber formation, but gave lower yield than non pre-sprouted tubers if there is no restriction of the growing season. The pre-sprouted tubers with stimulation of adventitious root formation method gave faster development and tuber initiation than conventional pre-sprouting. This can be usefull when the growing season is restricted or if early harvest is important. Besides, emergence was faster for the pre-sprouted treatments compared with the control. At harvest, the pre-sprouted treatments gave higher yield than the control. The new method for stimulating adventitious root formation developed here will substantially help growers to achieve earlier harvests (Hajman, 2012).
4. Effect of Catalase inhibitors on breaking potato dormancy
It has been established that hydrogen peroxide (H2O2) and thiourea, a catalase inhibitors research stimulated sprouting and shortened the period of dormancy (Bajji et al. ,2007). These two phenomena are accompanied by irreversible inhibition of catalase activity and an increase in peroxidase activity. Thiourea inhibits catalase, consequently surplus intracellular hydrogen peroxide stimulates superoxide dismutase which stimulates germination (Bajji et al., 2007). In the same contex, Suttle (2012) indicated that hydrogen peroxide applied on tubers converts to oxygen by monooxygenases involved in cellular respiration which leads to the synthesis of gibberellins implicated in breaking dormancy. Thereafter, mitochondrial membranes are important source of intracellular hydrogen peroxide, which ensures the breaking of dormancy of microtubers in normal conditions (Bhate and Ramasarma, 2009). In the same conditions, catalase uses 65% of the intracellular hydrogen peroxide; the rest (35%) is used by APX and glutathione peroxidases. Some researchs indicated that sprouting was optimal when tubers were treated with 60 mM of hydrogen peroxide, whereas at a lower concentration, sprouting is less stimulated. Besides, tubers treated with 250 mM thiourea had a maximum sprouting percentage and better sprouting capacity (Mani et al., 2012). So, hydrogen peroxide applied directly or indirectly (throughapplication of thiourea) stimulates sprouting only when it is applied at low concentrations. Hydrogen peroxide content was analysed during storage in the parenchyma tissues of tubers treated with hydrogen peroxide and thiourea (Mani et al., 2013). It has been concluded that the activity of antioxidant enzymes such as: superoxide dismutase, ascorbate peroxydase and catalase is increased when hydrogen peroxide or thiourea are applied (Pei et al., 2000). Consequently, hydrogen peroxide applied directly (20 mM) or indirectly (250 mM thiourea) would be sufficient to affect the cellular balance, leading to a cascade of biochemical reactions that are: calcium ion migration to the meristematic cells (Zabrouskov et al., 2002), increased respiration and increasing production of adenosine triphosphate (ATP) causing sprouting of tubers. These findings are in line with recent studies confirming that moderate oxygenation of cells through hydrogen peroxide causes an increase in intracellular Ca 2+ and an increase in the concentration of iron, and an active input in mitosis causing significant sprouting (Jones and Smirnoff, 2005). Besides, some studies suggest that hydrogen peroxide oxidises sprouting inhibitors naturally present in the plant, and that the hydrogen peroxide is itself a stimulator of sprouting rather than oxygen (Macheixet al., 2005). But, when the concentration of hydrogen peroxide increases (hydrogen peroxide (40 mM) or thiourea (500 and 750 mM), sprouting decreases (Mani et al., 2013). In this context, it was indicated that the accumulation of reactive forms of oxygen, specifically hydrogen peroxide is a major factor responsible for oxidative stress and functional decline in older cells (Halliwell, 2006). This accumulation of hydrogen peroxide implicates oxidation and several protein glycation: glycoxidation, desamidation, ubiquitation and conjugation with lipid peroxidation products (Desikan et al., 2005) which may lead to decrease in sprouting capacity of tubers and may even cause theirphysiological death (Grace, 2005). Many studies have been carried to explain the mechanism by which hydrogen peroxide breaks dormancy. Some of them propose that hydrogen peroxide regulates the expression of a number of genes whose expression products are involved in dormancy (Suttle et al., 2014). Others show that hydrogen peroxide acts at the genomic level, thereby inducing the expression of genes for the transition from the dormant phase to the sprouting phase (Leymarie et al., 2007). While genetic studies have established a relationship between application of hydrogen peroxide and activation of genes (ox GA 1, ox GA 2 and ox GA 3) implicated in the biosynthesis of gibberellins (Kloosterman et al., 2007). Biochemical studies performed in vitro postpone that treatment with hydrogen peroxide results in a decrease in the level of endogenous abscisic acid, due to deactivation of protein phosphatases 1 and ABI 1, ABI 2, 2C, involved in the biosynthesis of abscisic acid (Nicolas et al., 2003). Nonetheless, other studies suppose that hydrogen peroxide activates directly the production cytosolic calcium, protein kinases and phosphatases, which triggers the breaking of dormancy (Desikanet al., 2005). Besides, it was suggested that hydrogen peroxide may play a mediation role, and at a certain concentration, it stimulates abscisic acid and ethylene to induce potato sprouting (Bailly, 2004). From another point of view, hydrogen peroxide acts on the protein metabolism of the tuber (Fauconnier et al., 2002).
5. Release of apical dominance in potato tuber and programmed cell death
To study release of apical dominance in potato, natural dormancy release is initiated by tuber apical bud meristem sprouting characterized by apical dominance. Dormancy is shortened by treatments such as bromoethane, which mimics the phenotype of dormancy release in cold storage by inducing early sprouting of several buds simultaneously. Tuber apical bud meristem decapitation resulted in the development of increasing numbers of axillary buds, suggesting the need for natural dormancy release of each bud prior to control by the apical bud. Programmed cell death were identified in the tuber apical bud meristem during normal growth, and these were more expressed when apical dominance apical dominance was lost following bromoethane treatment. DNA fragmentation, led to gene expression of vacuolar processing enzyme1 (VPE1), and elevated vacuolar processing enzyme activity. Thereafter , vacuolar processing enzyme1 1 protein was semipurified from bromoethane-treated apical buds, and its endogenous activity was fully inhibited by a cysteinyl aspartate-specific protease-1-specific inhibitor N-Acetyl-Tyr-Val-Ala-Asp-CHO (Ac-YVAD-CHO) (Teper-Bamnolker et al., 2012).In addition, transmission electron microscopy revealed programmed cell death -related structural alterations in the tuber apical bud -meristem of bromoethane -treated tubers: a knob-like body in the vacuole, development of cytoplasmic vesicles, and budding-like nuclear segmentations. In fact ; treatment of tubers with bromoethane and then vacuolar processing enzyme1 inhibitor led to a faster growth and recovered apical dominance in detached and nondetached apical buds. We suppose that programmed cell death occurrence is associated with the weakening of tuber apical dominance, allowing early sprouting of mature lateral buds (Esmaielpour et al., 2011).
6. Genes in potato associated with tuber dormancy release
To identify differentially expressed genes in potato associated with tuber dormancy release, suppression subtractive hybridization was employed. Finally a total of 304 effective expressed sequence tags (ESTs) were obtained ultimately. They have been deposited in the EMBL\GenBank\DDBJ nucleotide sequence data libraries under accession numbers from JK483901 to JK484204. It has been shown that 45, 34 and 3 % ESTs were associated with binding, catalytic activity and signaling respectively, some of these ESTs were involved in plant dormancy breaking, while, 14 % of the ESTs did not show significant homology to any database proteins. Furthermore quantitative PCR (RT-qPCR) analysis of the expression patterns of 14 selectable transcripts indicated that 13 selected candidate genes were significantly up-regulated in the development process of tuber from dormancy to sprouting. Thereafter, a full length cDNA of ADP-ribosylation factor (ARF) gene was cloned and found it belonged to potato ARF1 gene. Furthermore, tissue specific expression analysis showed ARF1 expression level was the highest in tuber. RT-qPCR analysis of the expression profile of ARF1 gene from potato tuber dormancy to sprouting revealed that the ARF1 gene expression was significantly increased after tuber dormancy breaking, which indicated that it probably associated with tuber dormancy and sprouting (Liu et al., 2012).
7. Changes in the proteome and antioxidants during potato sprouting
During post-harvest storage of potato, a significative proteolysis of patatin occurred. At the same time, several glycolytic enzymes were up-regulated and cellular components influencing protein conformation and the response to stress were altered. The equilibrium between the 20S and 26S forms of the proteasome was modified, the 20S form that recycles oxidized proteins being up-regulated. In addition, two proteins belonging to the cytoskeleton were also differentially expressed during potato ageing. Many of these changes are also occured in an oxidative stress context, an approach focused on antioxidant compounds and enzymes as well as oxidative damage on polyunsaturated fatty acids and proteins was happened. These findings suppose that the potato tubers to maintain their radical scavenging activity until the end of the storage period as no accumulation of oxidative damage was noted (Delaplace et al., 2009).
8. Overexpression of bacterial 1-deoxy-D-xylulose 5-phosphate synthase gene in potato tubers enhance sprouting
Expression analysis of nine transgenic lines n potato tubers expressing a bacterial gene encoding 1-deoxy-D-xylulose 5-phosphate synthase (DXS) demonstrated that the at harvest, sprouting had already commenced in dxs-expressing tubers whereas in control lines no bud growth was evident until dormancy was released after 56–70 d of storage. The initial phase of bud growth in dxs tubers was followed by a lag period of ~56 d, before further elongation of the developing sprouts could be detected. Consequently, dxs expression results in the separation of distinct phases in the dormancy and sprouting processes. The major difference measured was an increase in the level of trans-zeatin riboside in tubers at harvest expressing dxs. Besides, compared with controls, in some dxs-expressing lines, tuber carotenoid content increased ~2-fold, with most of the increase accounted for by a 6–7-fold increase in phytoene (Morris et al., 2006).
9. Reactivation of meristem activity and sprout growth in potato
Certainly, the phytohormones cytokinins and gibberellins play important roles in releasing potato tuber dormancy and promoting sprouting, but their mode of action in these processes is still obscure. For this purpose, in vitro assay using excised tuber buds was established to study the dormancy-releasing capacity of gibberellins and cytokinins and show that application of gibberellic acid (GA(3)) is sufficient to induce sprouting. Nonetheless, treatment with 6-benzylaminopurine induced bud break but did not support further sprout growth unless gibberellins (3) was administered additionally. Thus, transgenic potato plants expressing Arabidopsis (Arabidopsis thaliana) gibberellins 20-oxidase or gibberellins 2-oxidase to modify endogenous gibberellins levels showed the expected phenotypical changes as well as slight effects on potato tuber sprouting. Furthermore, the isopentenyltransferase (IPT) from Agrobacterium tumefaciens and the Arabidopsis cytokinin oxidase/dehydrogenase1 (CKX) were exploited to modify the amounts of cytokinins in transgenic potato plants. IPT expression promoted earlier sprouting of in vitro tubers. In addition, CKX-expressing tubers exhibited a prolonged dormancy period and did not respond to gibberellins (3). This indicates the essential role of cytokinins in terminating tuber dormancy and pointed that gibberellins is not sufficient to break dormancy in the absence of cytokinins. Thus, gibberellins (3)-treated wild-type and CKX-expressing tuber buds were subjected to a transcriptome analysis that revealed transcriptional changes in several functional groups, including cell wall metabolism, cell cycle, and auxin and ethylene signaling, denoting events associated with the reactivation of dormant meristems (Hartman et al., 2011).
10. Sugar, the initial regulator of apical dominance
It has been established that the auxin indole-3-acetic acid is produced in the shoot tip and transported down the stem, where it inhibits bud growth. But, the initiation of bud growth after shoot tip loss cannot be dependent on apical auxin supply because bud release up to 24 h before changes in auxin content in the adjacent stem. Thereafter, sugars are rapidly redistributed over large distances and accumulate in axillary buds within a timeframe that correlates with bud release. Besides, artificially increasing sucrose levels in plants represses the expression of BRANCHED1 (BRC1), the key transcriptional regulator responsible for maintaining bud dormancy, and results in rapid bud release. An enhancement in sugar supply is both necessary and sufficient for suppressed buds to be released from apical dominance. These findingss suppose that apical dominance whereby the shoot tip's strong demand for sugars inhibits axillary bud outgrowth by limiting the amount of sugar translocated to those buds (Mason et al., 2014).
11. Starch-related Enzymes during Potato Sprouting
Activities of enzymes presumably involved in starch biosynthesis (ADP-glucose pyrophosphorylase, AGPase) and/or breakdown (starch phosphorylase, STP; amylases) decreased during the first stage of tuber dormancy, but remain unchanged at the time of dormancy breaking and sprouting. Nevertheless, ADP-glucose pyrophosphorylase activity decrease significantly during the dormant period and increase before noticable sprouting (Sergeeva et al., 2000 ; Golyanovskayaa et al., 2012 ). This increase was visible near the vascular tissue and at the apical bud, which showed a very intensive staining. Inaddition, the tissue distribution of starch phosphorylase was the same as for AGPase (Sergeeva et al., 2000 ; Sarkar, 2008). This may be du to the direct starch cycling : starch phosphorylase produces glucose-1-phosphate during starch breakdown, which can be directly used as a substrate by AGPase for starch synthesis. Besides, studies pointed an increase of the activity in sprouting tubers as compared to dormant tubers, with the highest expression levels observed around the apical buds (Kim et al., 2013). The presence of amylase activity at dormancy initiation and ADP-glucose pyrophosphorylase activity abundant at the sprouting stage indicate that starch was cycling throughout the entire dormancy period. But, the ADP-glucose pyrophosphorylase activity increased significantly before visible sprout growth and could be one of the first physiological determinants of dormancy breakage (Sergeeva et al., 2012).
12. Breaking dormancy of potato tubers
Potato tubers could be stored in controlled or rudimentary conditions. Under rudimentary conditions, such as store rooms of small farmers or houses; control of humidity and temperature could not be achieved. Respiration of tubers during storage and breakdown of dormancy during storage result in early sprouting and loss of nutritive value of tubers (Suhag et al., 2006). Dormancy also leads to physiological aging of tubers resulting in loss of weight and quality for fresh market tubers and also leads to yield losses if these tubers are used as seed tubers for planting (Yosuke et al., 2000; Suhag et al., 2006; Katundu et al., 2007). Dormancy release in potato tubers by external agents has many applications as dormancy breaking of seed tubers for export or local use, incorporation into seed multiplication programs and rapid post-harvest disease testing procedures. The method used to break dormancy depend on facilities and chemicals available as well as the genetic characteristics of the breeding materials and varieties to be treated (Ezekiel and Singh, 2003). The most common temperature treatments are the heat, and the cold shock plus heat. In the heat treatment, tubers are kept in a dark room at 18-250 °C until sprouting occurs. In the cold shock plus heat treatment, harvested tubers are placed in 40°C for 2 or more weeks and then held at 18-250C until sprouting occurs (Suttle, 2007). The chemicals used in dormancy breaking often fall within the following categories: respiratory inhibitors, sulfhydryls, anaesthetics or end products of glycolysis. In addition, some hormones (gibberellic acid, bromoethane) are used to improve sprout growth of seed potato. Rindite (a three component mixture containing ethylene chlorohydrin, ethylene dichloride and carbon tetrachloride) and GA3 have received considerable attention as routine dormancy breaking agents. Yet, rindite is extremely volatile, very dangerous and corrosive and should be handled with extreme care (Uwe, 2001 ; Muthoni et al., 2014).
13. Carbon dioxide and oxygen and ethylene
Dormancy release in potato buds and seeds by high concentrations of carbon dioxide and oxygen has been established by Pakizeh et al. (2014) , yet the specific physiological mechanisms are unknown .Tuber dormancy could be broken effectively with 40-60% CO2 and 20% O2 applied to tubers continuously for 3-7 d at 25 °C. Thereafter, an enhancement of this effect by high (20-80%) concentrations of O2. This may be due to a relatively O2-impermeable periderm while CO2 acted as a `metabolic regulator'. Nonetheless, some authors did not support the role of O2 as the major and sole factor that regulated dormancy (Qin et al., 2004). Other authors pointed that ethylene is more efficient to enhance tuber sprouting. In addition to its dormancy breaking effect, ethylene also influences the number of sites of sprouting of tubers, indicating an effect on apical dominance. Increased sprout numbers per tuber, following ethylene exposure, are reported by Streck et al. (2006) and Yoshoka et al. (2001).
14. Mint essential oil can induce or inhibit potato sprouting
It has been established that thermal fogging with mint (Mentha spicata L.) essential oil (MEO) inhibited sprouting in eight potato cultivars during large-volume 6-month storage: the tubers remained firm with 38% lower weight loss after 140 days of storage. This sprout-inhibitory action may be inhibited, as treated tubers washed with water resumed sprouting within days, with reduced apical dominance. Mint essential oil application led to a local necrosis of the bud meristem and an induce of axillary bud growth in the same sprouting eye few weeks later. Mint essential oil components analysis showed that 73% of its content is the monoterpene R-carvone. Potato tubers treated with synthetic R-carvone in equivalent dose, 4.5 microl l(-1), indicated an inhibitory effect similar to that of mint essential oil. However, 0.5 microl l (-1) of Mint essential oil or synthetic R-carvone catalyzed axillary sprouting in the tuber. Furthermore, R-carvone caused great damage to the meristem membrane at sprout-inhibiting, but not sprout-inducing doses, indicating different mechanisms. After 5 days' exposure to R- carvone, its derivatives transcarveol and neo-dihydrocarveol were found in buds of tubers treated with the inhibitory dose, suggesting biodegradation. These findings proposed the potential of mint essential oil vapor as an environmentally friendly alternative to CIPC in stored potatoes and as a research tool for the control of sprouting in plants (Teper-Bamnolker et al., 2010).
15. Cessation of potato dormancy by bromoethane
The cessation of potato dormancy results in rapid growth and may be associated with the production of nascent transcripts that encode for gene products controlling for cell division and growth during tuberization. Potato dormancy cessation occur normally or can be chemically induced using bromoethane. Thereafter, microarray analysis is used to detect changes in specific transcripts in response to dormancy cessation before a significant increase in cell division. Comparing normal dormancy cessation to dormancy cessation induced by bromoethane indicated a commonality in both up and downregulated transcripts. This comparison shows many transcripts that decrease as dormancy terminates are inducible by abscisic acid particularly in the conserved BURP domain proteins, which include the RD22 class of proteins and in the storage protein patatin. Also, transcripts associated with an increase in expression encoded for proteins in the oxoglutarate-dependent oxygenase family. These findings indicates that cessation of potato dormancy induced by bromoethane initiates transcript profiles similar to the natural processes that control dormancy (Campbell et al., 2008).
16. Anti-sprouting agents
Indesirable sprouting reduces the weight, the nutritional and processing quality of tubers and the number of marketable potatoes, this can cause being important economic losses during potatoes storage (Delaplace et al., 2008). Sprouting affect the internal composition of the tuber and destruction of edible material and changes in nutritional quality (De Carvalho and Da Fonseca, 2006). Many methods are available to control sprouting during storage. It has been established that postharvest application of isopropyl N-(3-chlorophenyl) carbamate (chloropropham; CIPC) can control potato tuberization. CIPC inhibits sprout development by interfering with cell division (Pringle et al., 2009). Yet, these agents are not environmentally acceptable. It’s recommanded to use natural products compounds such as essential oils as well as the pure compound derived from essential oils. Naturally occurring compounds could be used as anti-sprouting agents in potato seeds, based on the common idea that natural products are less harmful to the environment than chemical products (Frazier et al., 2004). Controling potato sprouting is strategic for a managing stored potato quality. Oil from the Muna plants was more effective than CIPC in inhibiting sprouting, fresh weight loss and the incidence of rotted tuber parts over a period of 120 days. In addition, the main components of the oil, including the monoterpenes alpha and beta-pinene and limonene and the oxygenated monoterpenes pulegone and menthone/isomenthone are effective in this regard. El-Aawady (2006) reported a method for inhibiting sprouting of tubers including the step of exposing tubers to the oxygenated monoterpenes. An antisprouting agent for potatoes based on the essential oil of caraway (rich in carvone) has been suggested (Suhag et al., 2006). Klinge et al. (2009) has reported that a combination of carvone and one or more fungicides inhibit succesufully potato sprouting. Benzaldehyde, salicylaldehyde and substituted benzoic acids have been shown to be inhibitory to the growth of plants, fungi and bacteria. Coleman et al. (2001) used several naturally occurring volatiles, thymol cuminaldehyde and salicylaldehyde. They were applied as volatiles or directly tubers stored at 22°C. They also found that thymol and volatiles have effectively inhibited sprouting relative to control of 98% sprouting. Besides, continuously bathed potato tuber to 1, 8-cineol, monoterpene of essential oil and ozone as alternatives to CIPC to control sprouting at ambient temperature. They found that 1, 8-cineol was as effective as CIPC in suppressing of sprouting (Klinge et al., 2009) .
17. Gamma irradiation inhibit potato sprouting
Application of Gamma irradiation is a well-known method to eliminate or inactivate the spoilage causes with no adverse effects on nutritional and sensory quality of foods (Bidawid et al., 2000). Also, Arvanitoyannis et al. (2008) indicated that irradiation on potato tubers inhibited sprouting and reduced the weight loss. Otherwise, the application of irradiation may be an alternative treatment for controlling undesirable changes in potatoes during longterm storage. Appropriate use of irradiation can extend shelf life, reduce the requirement of chemicals for preservation and pest control, produce sterilized products (controlling the microorganisms) that can be stored without low temperature applicatiob, delay the ripening of fruits and vegetables and decrease quality deterioration of stored tuber and bulb crops by preventing postharvest sprouting (Arvanitoyannis et al., 2009). Yet, it is primordial to find the optimum radiation level that can fulfill the preservation requirements without causing serious chemical alterations in the food, which would affect its organic acceptability and wholesomeness (Afshari, 2006). In this context, Frazier (2006) report that sprout suppression was achieved with doses of 40 to 50 Gy while higher doses caused undesirable increases in reducing sugars in the tubers. Doses between 50 and 150 Gy are recommended for sprout control of tubers and in dormant state shortly after harvest (Majd and Ardakani, 2003). Besides, Singh et al. (2009) suggested that irradiation during the dormancy period of tubers is the most effective for sprout control. Other study indicated that early irradiation and higher irradiation levels significantly decreased sprouting, percent weight loss and specific gravity of tubers. In addition, the loss of ascorbic acid and the contents of reducing and nonreducing sugars significantly increased by delay in irradiation whereas the content of sugars and ascorbic acid level decreased by irradiation. Besides, the loss of firmness became clearer during five months storage in non-irradiated tubers. Higher storage temperature (16°C) caused greater loss of ascorbic acid (20.34%).Consequently, tubers stored at 16°C showed many metabolic changes leading to sprouting, such as weight loss and changes in sugars and ascorbic acid contents (Rezaee et al., 2011). We precise that the 50 Gy irradiation treatment on the 10th day after harvest resulted in complete sprout inhibition of tubers at 8°C storage and 150 Gy dose while inhibiting sprouting at 16°C, caused greater loss of ascorbic acid. While tubers irradiated with later after harvest have greater loss of ascorbic acid in response to higher doses of irradiation and higher storage temperature (Menza, 2008).
18. Iodine-saturated atmosphere inhibit the sprouting of potato
Iodine-saturated atmosphere inhibit the sprouting of potato tubers. The iodine concentration in tuber tissues increased as a function of exposure length, and the onset of inhibition of sprouting was found to depend on tubers genotype. But, the transcription of polyphenol oxidases (EC 1.10.3.1 and EC 1.14.18.1) was undetectable in tuber peel, while in bud tissues featured an increase, followed by a decrease occurring simultaneously with the suppression of sprouting. Treating potato tubers with iodine vary the expression of polyphenol oxidases at the transcriptional level. In addition, polyphenol oxidase activity in buds is less correlated with the corresponding level of transcription. Besides, less differences were found among the enzyme isoforms expressed in buds as a function of length of exposure to iodine. These results suppose that the induction of polyphenol oxidases mRNAs transcription could probe the inhibition of sprouting by iodine in potato (Eolini et al., 2004).
19. Use of Isopropyl N-(3-chlorophenyl) carbamate (CIPC) and glyphosate as sprout suppressant
Isopropyl N-(3-chlorophenyl) carbamate (CIPC) is widely used as sprout suppressant in potato. It is applied in the form of aerosol on the stored potatoes as a Post-harvest application. In addition, using glyphosate as a pre-harvest foliar application on the potato crop was also recommanded. Moreover, glyphosate is safer in terms of lower mammalian toxicity with respect to oral LD50, contaminant level for human toxicity (long-term), acceptable daily intake limit (for human body) and acceptable residue limit (for human consumption). Yet, CIPC is more harmful to birds, fishes and the environment. In fact, metabolites of CIPC are more toxic and cytolytic in nature. Economic aspects such as cost of the chemical, application cost and ease of application also favor the glyphosate. Higher water solubility of glyphosate over the CIPC and its metabolites is also a point, as this can assist in reducing the residue levels of glyphosate either in processed or cooked potato tubers. It is so suggested the developing glyphosate as an alternative/supplementary to CIPC for its use as sprout suppressant on potatoes stored at 8–12 °C (Paul et al., 2014).
20. Conclusion
Tuber sprouting is controlled by phyotohormones, which play an important role in the initiation or inhibition of dormant tubers of potato. Sustained synthesis and action of endogenous ABA is required for both the initiation and maintenance of tuber sprouting, while ethanol and gibberillins may be involved in sprouting initiation. These phytohormones play major roles in the control of vascular development during sprouting. Reactive oxygen species including hydrogen peroxide are involved in breaking potato dormancy and sprouting, since they affect cellular balance, regulate the expression of genes whose expression products are involved in potato sprouting and act on the protein metabolism of the tuber. Inhibition of sprout growth is essentiel for the maintenance of tuber nutritional and processing qualities during long-term storage of potatoes. Actually, all chemicals registered for sprout suppression act through non-specific growth inhibition or tuber meristem injury. Suppression of tuber sprouting through extension of natural dormancy by manipulating endogenous hormones offers an attractive alternative to current suppression strategies; other strategies will have to be developed. As, multiple ABA gene expression and tuber encode the regulatory steps of tuber dormancy, identification of specific genes regulating ABA homeostasis in potato tubers during dormancy will require additional experimental evidence. Further research using both antisense and RNAi strategies is directed toward elucidating the role of individual gene members in these two families in the regulation of ABA levels during tuber sprouting.
21. References
Abid M. and Asghari B. (2006) .Role of Growth Promoting Substances in Breaking Potato (Solanum tuberosum L.) Tuber Dormancy. Journal of agriculture and social sciences, 2(3) :175-178.
Afshari, H.,(2006). Examination of Mechanical Properties and Impact Sensitivity of Potato Varieties and Mathematical Modeling of Tuber Damage Susceptibility. Ph.D. Thesis. Mechanics of Farm Machinery, Faculty of Agriculture, Sciences and Researches Branch, Islamic Azad University. Iran. P. 180.
Aksenova N., Sergeeva L., Konstantinova T., Golyanovskaya S., Kolachevskaya O. and Romanov, G., (2013). Regulation of potato tuber dormancy and sprouting. Russian Journal of Plant Physiology 60(3):301-312.
Arvanitoyannis I. S., Stratakos A. C. and Tsarouhas P. (2009). Irradiation Applications in Vegetables and Fruits: A Reviews. Crit. Rev. Food Sci. Nutr. 49(5): 427-462.
Arvanitoyannis I. S., Vaitsi O., Mavromatis A. (2008). Potato: A Comparative Study of the Effect of Cultivars and Cultivation Conditions and Genetic Modification on the Physio-chemical Properties of Potato Tubers in Conjunction with Multivariate Analysis Towards Authenticity. Crit. Rev. Food Sci. Nutr. 48(9): 799-823.
Bailly C., (2004). Active oxygen species and antioxidants in seed biology. Seed Sci. Res. 14: 93 -107.
Bajji M., Mhamdi M., Castiny F., Rojas-Beltran J. and Du Jardin P. , (2007). Catalase inhibition accelerates dormancy release and sprouting in potato (Solanum tuberosum L.). Biotechnologie Agrononie Society and Environment 11 (2): 121-131.
Bamberg JB, (2010) Tuber dormancy lasting eight years in the wild potato Solanum jamesii. Ame J Pot Res. 87: 226-228.
Bamnolker P.T., Buskila Y., Lopesco Y., Ben-Dor S., Saad I., Holdengreber V., Belausov B., Zemach H., Ori N., Lers A., and Eshe D.l., (2012). Release of Apical Dominance in Potato Tuber Is Accompanied by Programmed Cell Death in the Apical Bud Meristem. Plant Physiol.; 158(4): 2053–2067.
Barani M., Akbari N. and Ahmadi H., (2013). The effect of gibberellic acid (GA3) on seed size and sprouting of potato tubers (Solanum tuberosum L.). African Journal of Agricultural Research 8(29):3898-3903.
Bhate B. and Ramasarma T., (2009). Evidence for H2O2 as the product of reduction of oxygen by alternative oxydase in mitochondria from potato tubers. Archives of Biochemistry and Biophysics 486 (2):165-169.
Bidawid S., Farber J. M. and Sattar S. A., (2000). Inactivation of Hepatitis A Virus (HAV) in Fruits and Vegetables by Gamma- Irradiation. Int. J. Food Microbio. 57: 91-97.
Biemelt S., Tschiersch H. and Sonnewald U., (2004). Impact of altered Gibberellins metabolism on biomass accumulation, lignin biosynthesis, and photosynthesis in transgenic tobacco plants. Plant Physiology.135:254-265.
Campbell M ., Segear E., Beers L., Knauber D. and Suttle J., (2008). Dormancy in potato tuber meristems: Chemically induced cessation in dormancymatches the natural process based on transcript profiles. Functional Integrative Genomics 8: 317-328.
Carli C., Mihovilovich E., Yuldashev F., Khalikov D. and Kadian M. S., (2010). Assessment of dormancy and sprouting behavior of cip elite and advanced clones under different storage conditions in Uzbekistan. Potato Research 53: 313-323.
ChapmanH.W., (2006). Tuberisation in the potato plant. Physiologia Plantarum 11(2):215-224.
Christiansen J., Pederson H. and Feder C., (2006). Variations in physiological age among seed potato lots. Vol. 2: pp. 6-9. In: NJF - Seminar 386, Seed Potatoes: Physiological age, diseases and variety testing in the Nordic countries. (NJF, ed.): Nordic Association of Agricultural Science, 1-2 February, Sigtuna, Sweden.
Claassens M.M.J. and Vreugdenhil D., (2000). Is dormancy breaking of potato tubers the reverse of tuber initiation. Potato Research 43: 347-369.
Coleman W.K., G. Lonergan and P. Silk (2001). Potato sprout growth suppression by menthone and neomenthol, volatile oil components of Minthostachys, Satureja, Bystropogon and Mentha species. Am. J. Potato Res., 78: 345-354.
Delaplace P, Fauconnier ML, Sergeant K, Dierick JF, Oufir M, van der Wal F, America AH, Renaut J, Hausman JF, du Jardin P., (2009). Potato (Solanum tuberosum L.) tuber ageing induces changes in the proteome and antioxidants associated with the sprouting pattern. J Exp Bot. 60(4):1273-88. Desikan R., Hankock J. and Neill S., (2005). Reactive oxygen species as signalling molecules. pp. 169 -196. In: Smirnoff, N. (Ed.). Antioxidants and reactive oxygene species in plants. Oxford, UK:Blackwell Publishing.
Delaplace P., Y. Brostaux M.L. Fauconnier and P. du Jardin, (2008). Potato (Solanum tuberosum L.) tuber physiological age index is a valid reference frame in postharvest ageing studies. Postharvest Biol. Technol., 50: 103-106.
De Carvalho, C.C.C.R. and M.M.R. Da Fonseca, (2006). Carvone: Why and how should one bother to produce this terpene. Food Chem., 95: 413-422.
Eolini F, Hochkoeppler A, Credi A, Rodríguez AG, Poggi V., (2004). Polyphenol oxidase expression in potato (Solanum tuberosum) tubers inhibited to sprouting by treatment with iodine atmosphere. Phytochemistry. 65(15):2181-7.
Esmaielpour B. , Saeid Hokmalipour S., Jalilvand P. and Ghobad Salimi G., (2011). The investigation of paclobutrazol effects on growth and yield of two potato (Solanum tuberosum) cultivars under different plant density. Food, Agriculture and Environment . 9(3&4), 289-294.
Ezekiel R, Singh B (2003) Influence of relative humidity on weight loss in potato tubers stored at high temperatures. Indian J Plant Physiol. 8: 141-144.
Fauconnier M., Rojas-Beltran J., Delcarte J., Dejaeghère F., Marlier M. and Du Jardin P., (2002). Lipooxygenease pathway and membrane permeability and composition during storage of potato tubers (Solanum tuberosum L. cv. Bintje and Desirée) in different conditions. Plant Biology 4: 77-85.
Frazier M.J., N. Olsen and G.E. Kleinkopf, (2004). Organic and alternative methods for potato sprout control in storage. CIS 1120, University of Idaho, College of Agriculture and Life Sciences, Idaho, USA.
Frazier M. J., Kleinkopf G. E., Brey R.R. and Olsen N.L., (2006). Potato Sprout Inhibition and Tuber Quality After Treatment with High-energy Ionizing Radiation. Am. J. Potato Res. 83: 31-39.
Golyanovskayaa A. , L. I. Sergeevaa , and G. A. Romanova, (2012) . Hormonal Regulation of Tuber Formation in Potato Plants N. P. Aksenovaa , T. N. Konstantinovaa , S. Russian Journal of Plant Physiology,, Vol. 59, No. 4, pp. 451–466.
Grace S. , (2005). Phenolics as antioxidants. pp. 141- 168. In: Smirnoff, N. (Ed.). Antioxidants and reactive oxygene species in plants. Oxford, UK : Blackwell Publishing.
Hagman J., (2012). Different pre-sprouting methods for early tuber harvest in potato (Solanum tuberosum L.). Acta Agriculturae Scandinavica, Section B — Soil & Plant Science 62, (2).
Halliwell B., (2006). Reactive species and antioxidants. Redox biology is a fundamental theme of aerobic life. Plant Physiology 141: 312 - 322.
Hartmann A, Senning M, Hedden P, Sonnewald U, Sonnewald S., (2011). Reactivation of meristem activity and sprout growth in potato tubers require both cytokinin and gibberellin. Plant Physiol.155(2):776-96.
Hay R.K.M. and Porter J.R., ( 2006). The Physiology of Crop Yield. Blackwell, Oxford. 314 pp.
JonesM. and Smirnoff N., (2005). Reactive oxygen species in plant development and pathogen
defence. In: Smirnoff, N. (Ed.). Antioxidants and reactive oxygene species in plants. Oxford, UK: Blackwell Publishing. pp.197 -214.
Kaiser H, Richter U, Keiner R, Brabant A, Hause B, Dräger B., (2006). Immunolocalisation of two tropinone reductases in potato (Solanum tuberosum L.) root, stolon, and tuber sprouts. Planta, 225(1) 127-137.
Katundu M.G.C., S.L. Hendriks J.P. Bower, M. Siwela, (2007). Effects of traditional storage practices of small-scale organic farmers on potato quality. J. Sci. Food Agric., 87:1820-1825.
Kim JI, Baek D, Park HC, Chun HJ, Oh D-H, Lee MK, Cha J-Y, Kim W-Y, Kim MC, Chung WS, Bohnert HJ, Lee SY, Bressan RA, Lee S-W, Yun D-J (2013) Overexpression of Arabidopsis YUCCA6 in potato results in high-auxin developmental phenotypes and enhanced resistance to water deficit. Mol Plant 6: 337–349.Klinge K.M. and R.E. Palomino, (2009). Muna (sp. Minthostachis mollis) essential oil, as a natural alternative to control potato sprouting tested under different storage conditions. Proceedings of the 15th Triennial International Society for Tropical Root Crops Symposium, November 2-6, 2009, Lima, Peru, pp: 126-130.Kloosterman B., Navarro C., Bijsterbosch G., Lange T., Prat S., Visser R. and Bachem C., (2007). StGA2ox1 is induced prior to stolon swelling and controls GA levels during potato tuber development. Plant Journal 52:362 - 373.
Leymarie J., Bruneaux E., Gibot Leclerc S. and Corbineau F., (2007). Identification of transcripts potentially involved in barley seed germination and dormancy using cDNA AFLP. Journal of Experimental Botany 58:425- 437.
Liu B, Zhang N, Wen Y, Si H, Wang D., (2012). Identification of differentially expressed genes in potato associated with tuber dormancy release. Mol Biol Rep. 39(12):11277-87.
Macheix J., Fleuriet A. et Jay-Allemand C., (2005). Les composés phénoliques des végétaux.Un exemple de métabolites secondaires d’importance économique.Lausanne: Presses polytechniques et universitaires romandes.
Majd F. and Ardakani M. R., (2003). Nuclear Techniques in Agricultural Sciences. Tehran University Press. 330-352.
Mani F., (2012). Contol of dormancy of microtubers and tubers of potato (Solanum tuberosum L.). PhD. Thesis, High Agronomic Institut, Chott Mariem, Tunisia. 69pp.
Mani F., Bettaieb T., Doudech N. and Hannachi C., (2013). Effect of hydrogen peroxide and thiourea on dormancy breaking of microtubers and field-grown tubers of potato. African Crop Science Journal 21(3): 221- 234.
Mason MG, Ross JJ, Babst BA, Wienclaw BN, Beveridge CA., (2014). Sugar demand, not auxin, is the initial regulator of apical dominance. Proc Natl Acad Sci U S A. 111(16):6092-7.
Mehta R.A., T. Cassol, N. Li, N. Ali, A.K. Handa and A.K. Mattoo, (2002). Engineered polyamine accumulation in tomato enhances phytonutrient content, juice quality and vine life. Nat. Biotechnol., 20: 613–8.
Menza, Katana MwalimuURI , (2008). Methods for breaking dormancy of potato seed tubers (solanum tuberosum l.) : http://erepository.uonbi.ac.ke:8080/xmlui/handle/123456789/18975.
Morris WL, Ducreux LJ, Hedden P, Millam S, Taylor MA., (2006). Overexpression of a bacterial 1-deoxy-D-xylulose 5-phosphate synthase gene in potato tubers perturbs the isoprenoid metabolic network: implications for the control of the tuber life cycle. J Exp Bot. 57(12):3007-18.
Muthoni J., Kabira J., Shimeli H. and Melis R. , (2014). Regulation of potato tuber dormancy. AJCS 8(5):754 -759.
Nicolas G., Bradford K., Côme C. and Pritchard H., (2003). The biology of seeds: Recent research advances. Wallingford: CABI Publishing. pp. 211 - 216.
Oliveira J.S., Moot D., Brown H.E., Gash A. and Sinton S., (2012). Sprout development of seed potato tuber after different storage conditions Agronomy New Zealand 42.
Pakizeh M., A.A. Kuliev, Z.M. Mammadov, M. Ardjmand, M. Hasani., (2014). Investigation of gas composition (CO2, N2, O2 mixture) on dormancy of potato tuber. J. Appl. Sci. & Agric., 9(6): 2351-2355 .
Paul V., Rakesh Pandey, R. Ezekiel, Kumar D. , (2014). Potential of glyphosate as a sprout suppressant for potato (Solanum tuberosum L.) tubers during storage. Indian Journal of Plant Physiology. 19 (4) :293-305.
Pei Z., Murata Y., Benning G., Thomine S., Klusener B., Allen G., Grill E. and Schroeder J., (2000). Calcium channels activated by hydrogen peroxide mediate abscisicsignalling in guard cells. Nature 406:731 -734.
Pringle B.C. Bishop and R. Clayton, (2009). Potatoes postharvest. CAB International, UK. file:///C:/Documents%20and%20Settings/Administrator/Desktop/PotatoesPostHarvest.pdf.
Qin L., Y. Liu T. Wang S.J. Wei M.L. Block B., Wilson B., Liu, J.S. Hong, (2004). NADPH oxidase mediates lipopolysaccharide-induced neurotoxicity and proinflammatory gene expression in activated microglia. J Biol Chem, 279: 1415-1421.
Rezaee M., M. Almassi, A. Majdabadi Farahani, S. Minaei and M. Khodadadi., (2011). Potato Sprout Inhibition and Tuber Quality after Post Harvest Treatment with Gamma Irradiation on Different Dates. J. Agr. Sci. Tech. Vol. 13: 829-842-829.
Roy M. and Wu R.,, (2001). Arginine decarboxylase transgene expression and analysis of environmental stress tolerance in transgenic rice. Pl. Sci., 160: 869–75.
Sarkar D., (2008). The signal transduction pathways controlling in planta tuberization in potato: an emerging synthesis. Plant Cell Rep.27(1):1-8.
Sergeeva LI, de Bruijn SM, Koot-Gronsveld EAM, Navratil O, Vreugdenhil D, (2000) Tuber morphology and starch accumulation are independent phenomena: Evidence from ipt-transgenic potato lines. Physiol Plant 108: 435–443
Sergeeva L.I.; Claassens M.M.J.; Jamar D.C.L.; Plas L.H.W,. van der; Vreugdenhil, D. (2012). Starch-related Enzymes during Potato Tuber Dormancy and Sprouting. Russian Journal of Plant Physiology, 59 (4): 556-564.
Singh J. and Kaur L., (2009). Advances in Potato Chemistry and Technology. Academic press, PP. 348-355.
Sonnewald,S. and Sonnewald U., (2014). Regulation of potato tuber sprouting. Planta 2014 J 239(1):27-38.
Streck N.A et al., (2006) .Simulating the development of field grown potato (Solanum tuberosum L.)-AGMET-3633; No of 11.
Struik P. and Wiersema S., (1999). Seed potato technology. CSIRO, Wageningen, The Netherlands. pp. 350-352.
Struik P.C., (2006). Physiological age of the seed potato. Vol. 2: pp. 3-5. In: NJF -Seminar 386, Seed Potatoes: Physiological age, diseases and variety testing in the Nordic countries (NJF, ed.): Nordic Association of Agricultural Science, 1-2 February, Sigtuna, Sweden.
Suhag M., B.K. Nehra N. Singh and S.C. Khurana, (2006). Storage behaviour of potato under ambient condition affected by curing and crop duration. Haryana J. Hortic. Sci., 35: 357-360.Suttle J., (2004). Physiological regulation of potato tuber dormancy. American Journal of Potato Research 81: 253-262.
Suttle JC, (2007) Dormancy and sprouting.p.287-309. In: Vreugdenhil D (ed) Potato biology and biotechnology. Advances and perspectives. Elsevier.
Suttle J., Suzanne R. Abrams, Luis De Stefano- Beltrán L,. and Huckle L., (2012). Chemical inhibition of potato ABA-8'-hydroxylase activity alters in vitro and in vivo ABA metabolism and endogenous ABA levels but does not affect potato microtuber dormancyduration. Journal of Experimental Botany (63 (15): 5717-5725.
Suttle JC, Huckle LL, Lu S, Knauber DC., (2014). Potato tuber cytokinin oxidase/dehydrogenase genes: biochemical properties, activity, and expression during tuber dormancy progression. J Plant Physiol. 171(6):448-57.
Tiburcio A.F., T. Altabella and Masgrau C., (2002) . Polyamines. In: Bisseling, T. and J. Schell, (eds.), New Developments in Plant Hormone Research. Springer-Verlag, New York.
Uwe S , (2001) Control of potato tuber sprouting. Trends in Plant Science 6: 333-335.
Vreugdenhil D. and Struik P.C., (2006). An integrated view of the hormonal regulation of tuber formation in potato (Solanum tuberosum L.). Physiologia Plantarum 75(4):525-531.
Yoshioka H., K. Sugie H-J., Park H., Maeda N., Tsuda K., Kawakita N., Doke, (2001). Induction of plant gp91 phox homolog by fungal cell wall, arachidonic acid, and salicylic acid in potato. MolecularPlant–Microbe Interactions., 14: 725-736.
Yosuke,M., K.F. Yaptenco, N. Tomohiro, S. Toshiro, S. Hiroaki, M. Shinji, T. Katsumi, (2000). Property changes in potato tubers (Solanum tuberosum L.) during cold storage at 0 oC and 10 oC. Food Pre. Sci., 26: 153-160.
Zabrouskov V., Kumar G., Sychalla J. and Knowles N., (2002). Oxidative metabolism and the physiological age of seed potatoes are affected by increased -linolenate content. Physiologia Plantarum 116:172-185.